Fish Health Management
Fisheries Victoria Research Report Series - No. 32 December 2005
Executive Summary
Farming Murray cod (Maccullochella peelii peelii) is an expanding industry within Australia. Although the production of fingerlings for stock enhancement purposes is well-established with fingerlings being commercially available since early 1980's, growout of Murray cod for human consumption under aquaculture conditions has only recently been undertaken.
Disease outbreaks have long been recognised as a significant constraint to aquaculture production and economic viability. Disease-induced checks to growth and associated mortalities have profound impacts on future production. In order to ensure sustainable growth and production of the Murray cod farming industry, it is imperative that farmers be familiar with the common diseases and conditions that affect the health of their stock, and employ sound management strategies that reduce the incidence and severity of factors affecting fish health. Although there are numerous articles and general text books that deal with fish diseases in aquaculture, there are however, few that focus on health issues of Murray cod.
The objective of these guidelines is to provide fish health information to Murray cod fish farmers. These guidelines describe the common diseases, parasites and disorders that affect farmed Murray cod, and outline strategies to manage and maintain the health and well-being of Murray cod in aquaculture facilities. Above all these guidelines aim to promote the concept that prevention is far better than treatment.
Introduction
Fish health and aquaculture
Disease outbreaks have long been recognised as a significant constraint to aquaculture production and economic viability. A wide range of pathogens (viruses, bacteria, parasites etc), environmental factors (water quality, etc) and even husbandry factors have caused heavy losses in aquaculture facilities (Sarig 1971; Humphrey and Langdon 1985; Brown 1993; Noga 2000). Often these factors are linked in disease outbreaks. For example, a decline in water quality associated with poor husbandry practices may lead to an increase in the incidence of bacterial infections. Not only can disease outbreaks inflict heavy mortalities of stock, but fish in poor health have considerably lower growths rates which increases the time and costs to grow them to a marketable size. Some diseases can disfigure or render the fish unsightly which reduces their marketability.
There is an increasing trend towards intensification of aquaculture production especially through the use of recirculating aquaculture systems (RAS). The advantages of RAS include compactness (small footprint), biosecurity, high density production and their ability to control the environment in which the animals are reared. RAS are generally more environmentally friendly than other types of aquaculture systems due primarily to their reduced water usage and waste discharge. By their nature, RAS also offer a degree of biosecurity from the external environment, which, if properly managed, can reduce risk of disease outbreak in stock. Not surprising, RAS are attracting immense interest in Australia and the number of systems in operation continues to grow substantially along with the range of species being cultured using this technology (Larkin 2000). With intensification and increased stocking densities of fish, transmission of infectious diseases, when they occur, is often more rapid and devastating in terms of mortalities. Consequently, reducing the incidence and severity of diseases in intensive systems is critical to maintaining production performance.
A major source of pathogens to intensive aquaculture facilities is the water supply, especially if fish are present in that water.
Another important source of pathogens is new fish stock from other facilities, both these sources need to be appropriately screened and treated to ensure that introduction of pathogens to aquaculture facilities is minimised and biosecurity maintained.
Murray cod aquaculture
Farming Murray cod (Maccullochella peelii peelii) is an expanding industry within Australia, particularly in eastern states. Although the production of fingerlings for stock enhancement purposes is well-established with fingerlings being commercially available since early 1980's, growout of Murray cod for human consumption under aquaculture conditions has only recently been undertaken (Ingram 2000; Ingram and De Silva 2004). The industry currently employs a range of fish holding and culture facilities and husbandry techniques from earthen ponds for holding broodfish and extensive growing of fry and fingerlings under ambient conditions to plastic and fibreglass tanks for intensive grow-out and purging under environment controlled conditions. However, the majority of table-size Murray cod are grown in RAS.
There are numerous articles and general text books that deal with fish diseases in aquaculture, and there have been several key fish health workshops and associated publications undertaken within Australia that provide information on the identification, treatment and management of diseases (Humphrey and Langdon 1985; Bryden 1988; Munday 1990; 1992). However, few focus on health issues of Murray cod. Rowland and Ingram (1991) described the parasitic and fungal diseases of Murray cod, golden perch and silver perch, and a brief overview of diseases of Murray cod was given by Missen and Dobson 2000. At a national level, a strategic plan for aquatic animal health ('AQUAPLAN') and an aquatic animal disease veterinary emergency plan ('AQUAVETPLAN') have been developed (Anon 1999; Bernoth et al. 1999).
Objectives
Minimising stress from disease outbreaks and therapeutic treatments to maximise not only survival but also long term growth is critical to the Murray cod aquaculture industry. Disease induced checks to growth and associated mortalities at key physiological development stages have profound impacts on future production. In order to ensure sustainable production of the Murray cod farming industry, and to be competitive within the Australian and global aquaculture/seafood market place, it is imperative that farmers be familiar with the common diseases and conditions that affect the health and well-being of their stock, and to employ sound health management strategies, which aim to reduce the incidence and severity of these diseases and conditions and to maintain the biosecurity of their facilities.
The objectives of these guidelines are to provide information to fish farmers on:
- common parasites, diseases and conditions that affect the health of Murray cod in aquaculture.
- strategies to manage and maintain the health and well-being of Murray cod in aquaculture facilities.
Above all these guidelines aim to promote the concept that prevention is far better than treatment.
Monitoring fish health
When sampling fish for health examinations it is important to establish a stringent fish health-monitoring program incorporating a regular regime of sampling fish. Maintaining a history of events associated with each holding facility (tank or pond) or batch of fish greatly facilitates disease diagnosis and long-term management. These records should include daily mortalities, health checks, water quality and treatments. The type of information that should be recorded and frequency of collection is summarised in Appendix I. Sampling should be frequent enough to ensure diseases are detected in time to reduce their impact on fish health and survival. In fry rearing ponds sampling should be undertaken at least weekly whereas in tanks daily checks should be undertaken. Since some species of external parasites, such as Trichodina, will leave their host shortly after death, it is important to examine fish, live or moribund, as soon as possible after collection.
Prior to sampling fish, where possible monitor the behaviour of the fish. These observations, which will assist in diagnosis, may include:
- loss of appetite,
- listlessness,
- gulping at the water surface,
- "flashing" or "rubbing",
- flaring of gill covers (opercula),
- Swimming action - loss of balance, position in water column, swimming with head up, swimming with head down, spiralling etc.
- Number of fish affected and number of mortalities.
Prior to undertaking any biopsy or autopsy procedures, care should be taken in ensuring that fish are handled in such a manor to reduce stress and or that slaughter is done humanely.
Two chemicals have been registered for use as anaesthetics in aquaculture, Aqui-S and benzocaine (http://www.apvmqa.gov.au/). Dose rates for both chemicals depend on the size of fish and level of sedation required. Use of these chemicals should follow the instructions provided by the supplier. These drugs are administered through the water and are taken up across the gills. Exposure time should be minimised where possible to avoid death from over exposure.
Once a fish has been removed and examined with the naked eye, observations of the physical appearance of the fish should be made. These include:
- Body shape hollow gutted, swelling.
- Skin colouration changes, blotchiness, "saddleback", presence of sores, necrosis, ulcers, spots, bleeding, loss of scales, changes in texture.
- Eyes swollen, cloudiness.
- Fins tattered, frayed, eroded.
- Gills pale, swollen, filaments fused together, filaments clubbed/swollen, filaments eroded, excess mucus, presence of debris.
Dissection instruments used in examining and dissecting fish include blunt and fine-pointed forceps, blunt and pointed probes, dissecting scissors preferably with pointed tips, scalpels and a range of scalpel blades. Also a notepad and pencil will be required to take notes. Various sized petri dishes or shallow plastic trays are useful for holding fish for examination. Tissue samples biopsied from fish, such as gill tissue, can also be observed under a dissecting microscope in a petri dish, but for higher magnifications, tissue samples and mucus scrapings should be mounted on microscope slides for examination with a compound microscope.
Often it is better to make observations on living animals because preservation can distort soft-bodied organisms. Observations of motion and morphology of live animals assists identification.
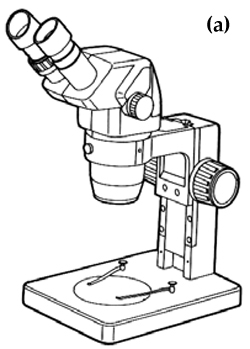
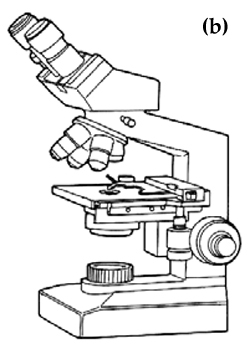
Small whole fish can be examined directly under a dissecting microscope (see below). However, for larger fish, samples of fish skin mucus, fins and gill tissue will need to be removed from the fish. A sample of mucus is collected by scraping the side of the fish with the back of a scalpel. The sample collected, skin cells, mucus and scales, can then be transferred to a drop of freshwater on a microscope slide. A coverslip may then be gently lowered onto the drop of water. Samples of gill tissue can be treated the same way. Whole gill arches can be removed from small euthanised fish whereas individual gill filaments can be snipped off a gill arch from larger sedated fish with a pair of scissors with minimal trauma. However, it is useful to examine the tissue with a dissecting microscope before adding a cover slip.
After dissections, all equipment should be disinfected to reduce the risk of spreading infectious pathogens.
Many of the pathogens of Murray cod are too small to be seen clearly by the naked eye and so special instruments and equipment are usually needed to locate and observe them in detail for identification purposes. Light microscopes provide the magnifications necessary to observe and identify parasites. Light microscopes are broadly separated into two types, dissecting microscopes and compound microscopes, based on their magnifying ability. The dissecting microscope (Figure 1a) is used for viewing specimens at low magnifications of between 5x and 60x. Combinations of lighting from beneath and above the specimen greatly improve viewing with a dissecting microscope. This type of microscope is suitable for most protozoan and metazoan parasites. However, for some protozoans, such as Ichthyobodo, and bacterial cells, a compound microscope (Figure 1b), which has magnifications of between 40x and 1,000x, is necessary. Compound microscopes with built-in illuminators and binocular eyepieces are more convenient than those with a separate lightsource or fitted with a single eyepiece. It is important to follow the guidelines supplied with these microscopes to ensure that settings and adjustments are correct to optimise viewing.
Diseases and parasites
A wide variety of diseases and parasites have been recorded from Murray cod, both in the wild and in captivity (Table 1). These include two viral, two bacterial, two fungal, nine protozoan, three trematode, five nematode, two copepod and two mite species, as well as several nonparasitic diseases and syndromes. However, only a small number of these have caused fish health problems and mortalities in aquaculture facilities, and some parasites have been recorded from wild fish only (Table 1). The most common parasites and diseases that affect the health of Murray cod in aquaculture facilities will be described here.
Species taxonomy
An arbitrary system of classification is used by biologists to name the different types of plants and animals, according to their degree of similarity or likeness to each other. This system of classification is extended to the pathogens that cause diseases. The "species" is the fundamental unit of this system, which represents a group of actually, or potentially interbreeding individuals that are reproductively isolated from other such groups. Each species receives two names; first is the generic name (eg. Maccullochella) which is common to all species of that "genus", and second is the specific name (eg. peelii) which is peculiar to the species in question. Traditionally the genus and species names are written in italics, and the genus starts with a capital letter while the species is written in lower case (eg. Maccullochella peelii). In some situations identification is taken below the level of species, ie. subspecies (eg. Maccullochella peelii peelii).
Viruses
Viruses can induce diseases resulting in important economic losses in aquaculture. In Australia, several fish viruses have been reported including epizootic haematopoietic necrosis virus (EHNV), an iridovirus, the picorna-like barramundi virus and a birnavirus from Atlantic salmon. To date, there has been one confirmed case of a virus infecting Murray cod. In February 2003, an iridovirus-like virus was observed from the spleen and kidneys and gills of juvenile Murray cod held in a recirculating aquaculture system (Lancaster et al. 2003). This infection resulted in 90% mortality in fingerlings (40-60 mm) and 25% mortality in larger fish (100-150 mm).
Juvenile Murray cod have also been experimentally infected with EHNV, which has caused mass mortalities of juvenile redfin perch in the wild (Langdon 1989). Under laboratory conditions infected Murray cod were found to be less susceptible to EHNV than other experimentally infected freshwater fish species, but were nevertheless potential carriers of the virus. EHNV is a potentially serious problem in aquaculture and as such is a notifiable disease both nationally and internationally (see Appendix 2). Consequently, this virus should be considered as a potential threat to the farming of Murray cod and precautions should be taken to prevent its introduction in aquaculture facilities.
Further information:
Langdon et al. 1986; 1988; Langdon 1989; Glazebrook et al. 1990; Crane et al. 2000; Lancaster et al. 2003.
Bacteria
Numerous bacterial organisms have been recorded from fish (Roberts 1982; Munday 1989; Carson 1990; 1992). Often, however, many of these occur as secondary infections and involve bacterial organisms which are normal inhabitants of the aquatic environment, but only cause disease following stress-induced factors such as parasitic infestation, changing environmental conditions (temperature, pollution etc.) and poor husbandry techniques. There are few published reports describing bacterial diseases of Murray cod (Table 1). However, in a survey of the Murray cod aquaculture industry (Ingram et al. 2004), 17% of respondents indicated experiencing problems with non-specific bacterial infections, often referring to them as cases of "fin rot", "tail rot" or "saddleback" diseases.
Table 1 Parasites and diseases recorded from Murray cod
PARASITE/DISEASE | SOURCE | FISH STAGE & SITE | REFERENCES | |
---|---|---|---|---|
VIRAL | Epizootic haematopoietic necrosis virus (EHNV) | Laboratory only | Internal organs | Langdon (1989) |
Hypertrophy iridovirus | Farmed | Internal organs | Lancaster et al. (2003) | |
BACTERIA | Myxobacteria | Wild | No data available | L. Ashburner pers com in Rowland and Ingram (1991) |
Aeromonas salmonocida nova (GUD) | Laboratory only | Body surface | R. Whittington pers com in Rowland and Ingram (1991) | |
FUNGI | Saprolegnia, & Achlya (Oomycete fungi) | Farmed | All life stages. Body surface & gills | Rowland and Ingram (1991) |
PROTOZOA | Chilodonella piscicola (= C. cyprini) | Farmed | Larvae, juveniles & adults. Gills | Ashburner and Ehl (1973) |
Chilodonella hexasticha | Farmed | Larvae, juveniles & adults. Body surface & gills | Rowland and Ingram (1991) | |
Epistylis | Farmed | Sub-adults. Body surface | MAFRI, Snobs Creek; Halliday and Collins (2002) | |
Goussia lomi | Farmed | Juveniles. Mucosa of intestine | Molnar and Rohde (1988) Philbey and Ingram (1991) | |
Ichthyobodo necator (= Costia necatrix) | Farmed | Larvae, juveniles & adults. Body surface & gills | Rowland and Ingram (1991) | |
Ichthyophthirius multifiliis (white spot) | Farmed | Larvae, juveniles & adults. Body surface & gills | Rowland and Ingram (1991) | |
Myxosoma | Farmed | Juveniles & adults. Gills | Ashburner (1978) | |
Tetrahymena | Farmed | Juveniles. Body surface & gills | Rowland and Ingram (1991) | |
Trichodina | Wild & Farmed | Larvae, juveniles & adults. Body surface & gills | Rowland and Ingram (1991) | |
METAZOA | Clinostomum complanatum | Farmed | Juveniles. Body cavity & eye | Anon (2001) |
Diplostomum Spathaceum | Wild | Juveniles & adults. Lens of eye | Johnston and Angel (1941) | |
Stegodexamene watsoni | Wild | Intestine | Cribb (1988) | |
Capillaria murrayensis | Wild | Intestine | Johnston and Mawson (1940) | |
Contracaecum sp. | Wild | Mesentry & omentum | Johnston and Mawson (1940, 1947) | |
Goezia fluviatilis | Wild | Gill mucus & intestine | Johnston and Mawson (1940, 1947, 1951) | |
Hysterothylacium murrayense | Wild | No data available | Johnston and Mawson (1940) | |
Spinitectussp. | Wild | No data available | Johnston and Mawson (1940) | |
Ergasilus intermedius | Wild | Gills | Kabata (1992) (see also Ingram and Philbey 1999) | |
Lernaea sp. | Wild & farmed | Juveniles & adults. Body surface | Ashburner (1978); Rowland and Ingram (1991) | |
Histiostoma papillata | Farmed | Juveniles. Body surface & gills | Halliday and Collins (2002) | |
Hydrozetes sp. | Farmed | Juveniles. Body surface | D. Walters, pers comm in Halliday and Collins (2002) | |
OTHER | Fatty liver syndrome | Farmed | Juveniles. Liver | MAFRI, Snobs Creek |
Chronic erosive dermatopathy (CED) | Farmed | Juveniles & adults. Body surface | Humphrey et al. (2000); Trott (2000); Baily (2003, 2005) | |
Blue-sac syndrome | Farmed | Eggs and larvae | MAFRI, Snobs Creek, Gunasekera et al. (1998) | |
Hypoxia | Wild | McKinnon and Shepheard (1995) | ||
Gas bubble disease | Farmed | Larvae, juveniles & adults | MAFRI, Snobs Creek |
Cytophaga- and Flexibacter-like bacteria ("myxobacteria"), in particular Flexibacter columnaris , typically infect the gills, body surface and fins of fish, especially juveniles. Colonies of F. columnaris involve large numbers of bacteria arranged in long parallel rows. In severe infections the skin becomes eroded dorsally, which gives rise to the term 'saddleback' disease. These bacteria can also be associated with "fin rot" and "tail rot" diseases in which the fins become severely tattered and eroded. Acute infections by these bacteria can lead to mortalities.
Aeromanas salmonicida is responsible for furunculosis, a serious and important disease of farmed salmonids overseas. In Australia, Goldfish Ulcer Disease (GUD), which is caused by the atypical strain Aeromanas salmonicida nova, was first recorded from a goldfish farm in Victoria in 1974, and has since been reported from goldfish and carp in the wild, goldfish on other fish farms, and farmed silver perch. GUD is a notifiable disease in Victoria (see Appendix 2). A. salmonicida nova may infect fish that otherwise look normal in appearance, and may survive for several months in an aquatic environment in the absence of a fish host. Fish infected with GUD develop haemorrhagic lesions on the skin (Figure 2). Mortality rates in silver perch infected with GUD are low in the absence of infections from other pathogens. Murray cod are apparently resistant to GUD, but may act as carriers without showing clinical signs of its presence. A. hydrophila is usually associated with haemorrhagic septicaemia and has been isolated from several native fish species.
Mycobacterium and Pseudomonas species of water borne bacteria are cosmopolitan and ubiquitous, and are often isolated from sick fish. Pseudomonas is responsible for redspot disease in eels.
Biofilms form on all wet surfaces within aquaculture facilities. Indeed, bacterial biofilms in biological filters are responsible for nitrification processes. Biofilms may also contain pathogenic micro-organisms, which may also pose a health risk to humans through direct exposure and via processed fish products. Pathogenic bacteria, including Aeromonads, Vibrios, Yersinias and Bacillus cereus, have been isolated from recirculating aquaculture systems, but whether the presence of these bacteria pose a health risk is uncertain (King and Flick 2000).
Stages & sites infected:
Larvae, juveniles, sub-adults and adults. Gills and body surface, including fins, internal organs and tissues, depending on the bacterial pathogen involved.
Symptoms:
Fish cease feeding and become listless. Symptoms vary depending on the bacterial pathogen involved. External symptoms include discolouration and/or blotchiness of the skin, reddening of parts of the fins and body surface, haemorrhagic lesions or ulcers on the body surface and erosion of fins. Internal symptoms include haemorrhages on the visceral organs.
Diagnosis:
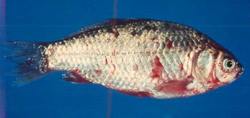
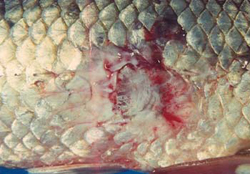
Bacterial cells can be observed with a compound microscope at high magnifications (> x400 magnification). However, identification of bacteria requires the skills of a specialist. If bacterial infections are suspected, contact a qualified veterinarian and prepare samples for submission (See Appendix 3). Identification of bacterial species and strains involves a variety of diagnostic methods including, culture using selective or specific enrichment culture media, staining, fluorescent antibody testing, enzyme-linked immunosorbant assay (ELISA), outer membrane proteins analysis, DNA fingerprinting and immunochemistry techniques.
Immediate Action:
Seek advice of a registered veterinarian.
Long-term management:
Most bacterial diseases arise from stressful conditions, frequently associated with poor husbandry and management practices. Improving these practices and taking an active approach to managing the health of stock can reduce the incidence and impact of bacterial diseases. In particular, maintain hygienic conditions, quarantine all new stock, avoid poor water quality, minimise unnecessary stress, manage other diseases, and avoid overcrowding and excess handling.
Comments:
Bacterial diseases are commonly treated with antimicrobial agents (antibiotics). Currently, however, there are no antimicrobial agents registered for use on aquaculture fish in Australia. Regardless, use of these restricted drugs must be done under the supervision of a qualified veterinarian, and should be confined to emergency situations only. In extreme bacterial disease outbreaks, it may be necessary to destroy infected stock and eliminate the responsible pathogens by sterilisation of ponds, tanks and equipment.
Use of antibiotics to treat diseases should be undertaken with extreme caution as bacteria have the ability to develop and transfer drug resistance. The occurrence of multiple antibiotic resistance by bacterial fish pathogens is becoming more evident in farmed fish, especially in areas where antibiotics are widely and indiscriminately used (Brown 1989; Dixon 1994; Alderman and Hastings 1998). Fortunately, as the fish farming industry has grown, effective vaccines are being developed for some bacterial pathogens and fish species, and are becoming more readily available to replace the use of antibiotics for control of bacterial diseases.
Further information:
Whittington et al. 1987; Munday 1989; Carson 1990; 1992; Callinan and Rowland 1995.
Fungi
Oomycete fungi (Saprolegnia)
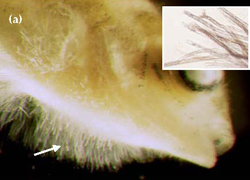
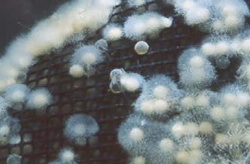
In a survey of the Murray cod aquaculture industry (Ingram et al. 2004), 19% of respondents had encountered fungal infections on stock. Most fungal infections recorded from fish are caused by species belonging to the oomycete fungi, in particular Saprolegnia, Achlya and Aphanomyces. Diseases caused by these fungi are collectively called "saprolegniasis". Oomycete fungi, which are ubiquitous and common in moist environments, are rarely considered to be primary disease pathogens, but are more often classed as saprophytic opportunists that will readily colonise damaged tissues infected by bacteria or parasites, and dead or decaying matter. Fungal growths appear as patches of white to whitish-grey cottonwool like growths, which are composed of numerous hyphae (Figure 3a). Murray cod eggs are particularly prone to attack by fungi (Figure 3b).
Stages & sites infected:
Eggs, larvae, juveniles, sub-adults and adults. Gills and body surface, including fins. Infects a wide variety of freshwater fish species.
Symptoms:
Fish cease feeding and become listless. Body surface develops off-white cottonwool like growths.
Diagnosis:
Visible examination of body surface, fins and gills for fungal hyphae (Figure 3), and fresh wet preparation of skin mucus and gill tissue for examination under a dissecting microscope.
Immediate Action:
Eggs:
Formalin at 1,000 ppm for 30 minutes daily until commencement of hatch. During hatching, reduce duration of treatments to 15 minutes daily. Ensure water is well aerated during treatment.
Larvae (tanks):
Salt at 5-7 g/L for 30 mins.
Juveniles (tanks):
Salt at 10g/L for 30-60 mins or 5g/L for 24-72 hours.
Formalin at 25-50 ppm for 60 mins (ensure water is well aerated).
Sub-adults & adults (tanks):
Salt at 10g/L for 60 minutes plus 5g/L for 24-72 hours.
Formalin at 50-150 ppm for 60-90minutes (ensure water is well aerated).
Fish in ponds:
No treatment recommended. Consult registered veterinarian.
Long-term management:
Improve husbandry practices and take an active approach to managing fish health. Maintain hygienic conditions and prevent accumulation of decaying matter. Remove dead eggs and animals, avoid poor water quality, reduce/avoid stressful episodes, control other diseases and avoid excessive handling.
Further information:
General fish disease text books. See also Neish and Hughes 1980; Rowland and Ingram 1991.
Epizootic ulcerative syndrome (EUS)
Epizootic ulcerative syndrome (EUS) has not been reported from Murray cod. However, since EUS is a severe and economically important disease affecting farmed freshwater fish it is listed as a notifiable disease in Australia (see Appendix 2), and significant outbreaks have already occurred in farmed silver perch in NSW (Figure 4). EUS is characterised by the presence of the fungus Aphanomyces that invades the body surface. Often, a range of other opportunistic organisms will invade the wounds as secondary agents, including bacteria and other fungal species. A range of both biotic and abiotic factors may predispose fish to infection by EUS. Natural outbreaks appear to be limited to latitudes between 35°N and 35°S. Optimal conditions for growth of Aphanomyces appears to be between 20°C and 30°C. Aphanomyces is spread with contaminated water and fish.
Stages & sites infected:
Wide range of freshwater and estuarine warmwater fish species in south-east Asia and north-eastern Australia. Juveniles, sub-adults and adults. Gills and body surface, including fins.
Symptoms:
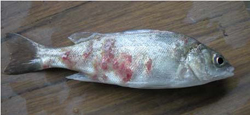
Reduction or loss of appetite and become lethargic. Often float near the water surface and/or swim with the head upwards. In the initial stages, body surface including head and fins develops pinhead-sized red spots. In later stages, spots may develop into 2-4 cm ulcers that may further expand in size to become open necrotic lesions (Figure 4). In extreme outbreaks mortalities may occur.
Diagnosis:
Histological sectioning of lesions and isolation of Aphanomyces from internal tissues.
Immediate Action:
Larvae (tanks):
Salt at 5-7 g/L for 30 mins.
Juveniles (tanks):
Salt at 10g/L for 30-60 mins or 5g/L for 24-72 hours.
Formalin at 25-50 ppm for 60 mins (ensure water is well aerated).
Sub-adults & adults (tanks):
Salt at 10g/L for 60 minutes plus 5g/L for 24 - 72 hours.
Formalin at 50-150 ppm for 60-90minutes (ensure water is well aerated).
Fish in ponds:
Remove all stock, dry and sterilise by liming. Consult registered veterinarian.
Disinfect all contaminated equipment.
Long-term management:
Improve husbandry practices and take an active approach to managing the health of stock. Maintain hygienic conditions, obtain stock from EUS free facilities and/or seek health certification, quarantine all new stock, avoid poor water quality and reduce/avoid stressful episodes.
Further information:
Callinan and Rowland 1995; Lilley et al. 1998; Callinan et al. 1999.
Protozoan parasites
Twenty eight percent of respondents to a survey of the Murray cod aquaculture industry (Ingram et al. 2004), had experienced diseases caused by protozoan parasites, particularly the ciliated ectoparasites Chilodonella, Trichodina and Ichthyophthirius multifiliis (white-spot), and the flagellated protozoan Ichthyobodo.
Ichthyobodo (Costia)
Ichthyobodo necator (Costia necatrix) (Figure 5) is a small flagellated protozoan (10-15 μm in length) which is an obligate parasite of fish. In crowded conditions, Ichthyobodo spreads rapidly. Each cells bears 2-4 flagella that are used for locomotion while not attached to the host. Ichthyobodo parasitises many species of fish and death from infestation is more likely to occur in larvae and juveniles than adults.
Stages & sites infected:
Larvae, juveniles, sub-adults and adults. Gills and body surface, including fins. Infests a wide variety of freshwater fish species.
Symptoms:
Fish cease feeding and become listless. Infected fish may "flash" and swim erratically. Heavily infested fish experience difficulty breathing. Skin may develop a bluish-white cloudy layer. The gills of heavily infested fish become clogged with mucus and the filaments may appear swollen.
Diagnosis:
Fresh wet preparation of skin mucus and gill tissue examined under a compound microscope (view at > x200 magnification). Ichthyobodo when alive and not attached to the host exhibit a characteristic jerky or "flicking" swimming motion. Ichthyobodo cells are teardrop-shaped (periform) and are about the same size as skin cells. The flagella are difficult to see.
Immediate Action:
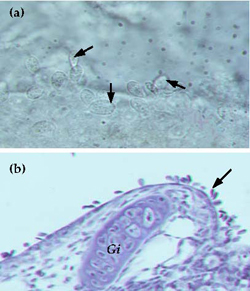
Larvae (tanks):
Salt at 5-7 g/L for 30 mins daily.
Juveniles (tanks):
Salt at 10g/L for 30-60 mins daily or 5g/L for 24-72 hours.
Formalin at 25-50 ppm for 60 mins (ensure water is well aerated).
Sub-adults & adults (tanks):
Salt at 10g/L for 60 minutes daily plus 5g/L for 24-72 hours.
Formalin at 50-150 ppm for 60-90minutes (ensure water is well aerated).
Fish in ponds:
No treatment recommended. Consult registered veterinarian.
Disinfect all contaminated equipment.
Long-term management:
Improve husbandry practices and take an active approach to managing the health of stock. Maintain hygienic conditions, quarantine all new stock, avoid poor water quality, reduce/avoid stressful episodes, control other diseases and avoid excess handling.
Further information:
General fish disease text books. Also see Becker and Kreier 1977; Rowland and Ingram 1991.
Ichthyophthirius (white spot)
Ichthyophthirius multifiliis is a large ciliated protozoan that has a complex lifecycle (Figure 6) which includes both free-living and parasitic stages. Fish parasitised by Ichthyophthirius are said to have "ich" or "whitespot". Successful treatment of this disease is greatly improved by understanding the lifecycle of Ichthyophthirius. The trophont stage is parasitic and burrows under the upper layer of the skin (epithelial tissue). Trophonts are large cells (0.05-1.0 mm) that are clearly visible to the naked eye as white spots on the skin of infested fish (Figure 7a). The trophonts, which are covered with many rows of cilia, constantly rotate slowly underneath the skin, and sometimes the distinctive horseshoe-shaped macronucleus is visible (Figure 7b & c). Trophonts may move from one host to another. Mature trophonts leave the host and become encysted tomonts. Within the cyst, reproduction occurs by binary fission and many tomites are formed. Following rupture of the cyst, tomites are released into the water to become actively swimming theronts that seek out a new host (Figure 6). Once finding a host they burrow beneath the skin and develop into trophonts. Ichthyophthirius is parasitic for about half its lifecycle. Because the trophonts are beneath the surface of the skin, many therapeutics are ineffective. Instead, control of Ichthyophthirius relies on destroying the free-living stages and preventing re-infection. The time taken between parasitising a host and leaving the host to reproduce is dependent on water temperature and takes less than 4 days at temperatures above 20°C, and up to 2 weeks at 15°C.
Stages & sites infected:
Larvae, juveniles, sub-adults and adults. Gills and body surface, including fins. Infests a wide variety of freshwater fish species..
Symptoms:
Fish cease feeding and become listless. Infected fish may "flash" and show signs of breathing difficulty. White spots (0.5- 1.0 mm) clearly visible on the body surface
Diagnosis:
Mature trophonts are visible to the naked eye on the body surface of infested fish. Confirm by fresh wet preparation of skin mucus and gill tissue examined under a dissecting microscope.
Immediate Action:
Due to the nature of the lifecycle, treatment regimes must extend over a full lifecycle of the parasite.
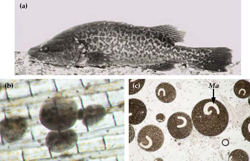
Larvae (tanks):
Salt at 5-7 g/L for 30 mins daily (up to 2 weeks)
Juveniles (tanks):
Salt at 10g/L for 30-60 mins daily or 5g/L for 24-72 hours (up to 2 weeks).
Formalin at 25-50 ppm for 60 mins (ensure water is well aerated).
Sub-adults & adults (tanks):
Salt at 10g/L for 60 minutes daily plus 5g/L for 24-72 hours (up to 2 weeks).
Formalin at 50-150 ppm for 60-90minutes (ensure water is well aerated).
Fish in ponds:
No treatment recommended. Consult registered veterinarian.
Disinfect all contaminated equipment.
Long-term management:
Improve husbandry practices and take an active approach to managing the health of stock. Maintain hygienic conditions, quarantine all new stock, sterilise influent water and reduce/avoid stressful episodes.
Comments:
Outbreaks of this parasite have caused mass mortalities on fish farms in Australia and overseas. Larvae and fry may die before trophonts are detected. All life stages (except eggs) of Murray cod are susceptible to infection by this parasite and mass mortalities have been recorded in aquaculture facilities.
Further information:
General fish disease text books. Also see Hoffman 1978; Rowland and Ingram 1991.
Chilodonella
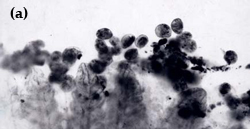
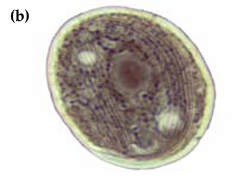
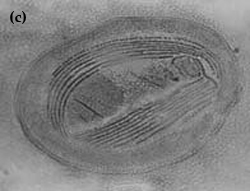
Chilodonella is a ciliated protozoan, which includes free-living species as well as facultative and obligate parasite species (Figure 8a and b). Species of Chilodonella are distinguished from other ciliated parasites by the typical gliding movement, the flattened and slightly distorted oval shaped body, the bottom surface is flat while the upper surface is slightly domed or vaulted. The upper surface has a series of ciliary rows (Figure 8c). Chilodonella are about 50-70 μm in length.
Stages & sites infected:
Larvae, juveniles, sub-adults and adults. Gills and body surface, including fins. Infests a wide variety of freshwater fish species.
Symptoms:
Fish cease feeding and become listless. Often show signs of breathing difficulty including flaring of opercula and swimming with the head upwards. Some infected fish may "flash". Skin becomes pale and may exhibit a white cloudiness. The gills of heavily infested fish are clogged with mucus and the filaments may appear swollen and fused together (Figure 8a).
Diagnosis:
Fresh wet preparation of skin mucus and gill tissue examined under a dissecting microscope (Figure 8a).
Immediate Action:
Larvae (tanks):
Salt at 5-7 g/L for 30 mins daily.
Juveniles (tanks):
Salt at 10g/L for 30-60 mins daily or 5g/L for 24-72 hours.
Formalin at 25-50 ppm for 60 mins (ensure water is well aerated).
Sub-adults & adults (tanks):
Salt at 10g/L for 60 minutes daily plus 5g/L for 24-72 hours.
Formalin at 50-150 ppm for 60-90minutes (ensure water is well aerated).
Fish in ponds:
No treatment recommended. Consult registered veterinarian.
Disinfect all contaminated equipment.
Long-term management:
Improve husbandry practices and take an active approach to managing the health of stock. Maintain hygienic conditions, quarantine all new stock, sterilise influent water and reduce/avoid stressful episodes.
Comments:
Outbreaks of this parasite have caused mass mortalities on fish farms in Australia and overseas. All life stages (except eggs) of Murray cod are very susceptible to infestation by these parasites and mass mortalities of larvae, juveniles and broodfish have been recorded.
Further information:
General fish disease text books. Also see Hoffman 1978; Rowland and Ingram 1991.
Trichodina
Trichodina is a ciliated protozoan that is distinguished from other parasites by its typical circular shape and active spinning action (Figure 9a). The body is disc or bowler-hat-shaped and the posterior end, which is fringed with a ciliary band, possesses an adhesive disc containing denticles (hook-like structures) (Figure 9b & c). Trichodina are 25-100 μm in diameter.
Stages & sites infected:
Larvae, juveniles, sub-adults and adults. Gills and body surface, including fins. Infests a wide variety of freshwater fish species.
Symptoms:
Fish cease feeding and become listless. Infected fish often "flash" and show signs of breathing difficulty, including flaring of opercula. Heavily infested fish may swim with the head upwards. Skin may become blotchy and exhibit a bluish-grey colouration. Fins of heavily infected juvenile fish become tattered. The gills of heavily infected fish become clogged with mucus and the filaments may appear swollen.
Diagnosis:
Fresh wet preparation of skin mucus and gill tissue examined under a dissecting microscope.
Immediate Action:
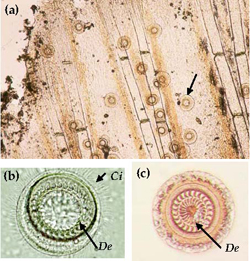
Larvae (tanks):
Salt at 5-7 g/L for 30 mins daily.
Juveniles (tanks):
Salt at 10g/L for 30-60 mins daily or 5g/L for 24-72 hours.
Formalin at 25-50 ppm for 60 mins (ensure water is well aerated).
Sub-adults & adults (tanks):
Salt at 10g/L for 60 minutes daily plus 5g/L for 24-72 hours.
Formalin at 50-150 ppm for 60-90minutes (ensure water is well aerated).
Fish in ponds:
No treatment recommended. Consult registered veterinarian.
Disinfect all contaminated equipment.
Long-term management:
Improve husbandry practices and take an active approach to managing the health of stock. Maintain hygienic conditions, quarantine all new stock and reduce/avoid stressful episodes. Sterilise influent water.
Comments:
Mortalities are more likely to occur in larvae, fry and fingerlings that are heavily infested. Adults may carry the parasite without apparent effect. Infestations often associated with poor water quality.
Further information:
General fish disease text books. Also see Hoffman 1978; Rowland and Ingram 1991.
Sessile peritrichs
Sessile peritrichs (Apiosoma, Epistylis, Scyphidia, etc) are ciliated protozoans that are mostly commensal in habit, attaching to hard surfaces by means of a holdfast or stalk. Depending on species, sessile peritrichs may occur as individuals (eg Apiosoma) or in colonies (eg Epistylis [sic Heteropolaria.]). Some species possess a stalk (Epistylis) (Figure 10), which may or may not be contractile. Most sessile peritrichs are filter-feeding bacterivores.
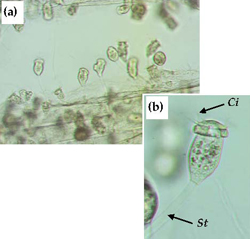
Infestations of fish by sessile peritrichs are uncommon, but when they occur it is usually associated with poor water quality and high organic loading. Juveniles, sub-adults and adults are affected, and the fins, body surface and gills are the sites most affected. Mortalities are more likely to occur in fry and fingerlings that are heavily infested. Treatments for control of sessile peritrichs are similar to those used to control other external protozoan parasites. Improve husbandry practices and take an active approach to managing the health of stock. Maintain hygienic conditions, improve water quality to reduce organic loading and reduce/avoid stressful conditions.
Other protozoan parasites
A range of other parasitic protozoan species has been recorded from freshwater fish, including ciliates, flagellates, coccidians, sporozoans, myxozoans, microsporidians and amoebae. But these are less commonly encountered, or are less threatening to fish than those species described previously. The coccidian Goussia lomi has been recorded from the stomach lining of juvenile Murray cod (Table 1). Cysts of Myxosoma have been observed on the gills of Murray cod and the ciliate Tetrahymena on the body surface and gills of juveniles (Table 1). There have been unconfirmed, anecdotal reports of outbreaks of Oodinium causing mortalities in intensively farmed Murray cod.
Further information:
General fish disease text books. Also see Becker and Kreier 1977; Hoffman 1978; Beumer et al. 1982; Paperna 1991; El-Matbouli et al. 1992.
Metazoan parasites
Monogenean and digenean trematodes (flukes)
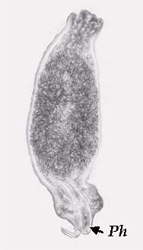
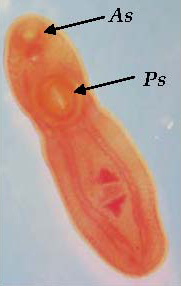
Monogenean trematodes (flukes) mostly parasitise the body surface, and gills of fish. Dactylogyrids and gyrodactylids are the two most common monogeneans that are important parasites in aquaculture. Dactylogyrids that possess eyespots and lay eggs are found predominantly on the gills of fish (Figure 11), whereas gyrodactylids lack eyespots, bear live young and are usually found on the skin. Both groups attach to the host by means of hooks, anchors and suckers. Monogeneans are about 0.3-2.0 mm in length. Symptoms of the presence of these parasites include fraying of the fins, excess mucus production, ulcerations, "flashing", flaring of the opercula and inflammation of the gill filaments. Mass mortalities attributed to monogeneans have been reported in juvenile fish overseas (Thoney and Hargis 1991). The incidence of monogeneans on farmed fish is reduced by maintenance of good husbandry and management practices, quarantining of all new stock and filtration of inlet water.
Digenean trematodes are mostly internal parasites that infest all types of tissues and organs. Mature stages possess one anterior and one ventral sucker. Digeneans have a complex lifecycle, which may include several hosts including both aquatic invertebrates and vertebrates. Both metacercariae (juveniles) and adult digeneans parasitise fish. Clinostomum complanatum (Figure 12) has been recorded from the body cavity and eyes of farmed juvenile Murray cod in southern Queensland (Anon 2001, W. Townsend, pers comm). These parasites (4-5 mm long) are visible to the naked eye as whitecoloured spots amongst the organs in the body cavity. Although this report did not indicate that significant mortalities occurred as a result of this infestation, parasitism of farmed fish by C. complanatum is a concern as the presence of the highly visible grub is unsightly and may cause rejection of fish at the market. Further, there are numerous reports of humans being infected by Clinostomum following ingestion of uncooked freshwater fish harbouring the parasite (Isobe et al. 1994; Coulibaly et al. 1995).
Controlling and preventing parasitism by digeneans, such as Clinostomum, involves breaking the lifecycle by the reduction or eradication of other hosts, especially aquatic snails.
Nematodes (roundworms)
A typical nematode is elongate and cylindrical in cross-section. Both the larval and adult stages of nematodes parasitise fish, and numerous species have been reported from native fish species (Beumer et al. 1982), including Spinitectus from Murray cod (Figure 13). Nematodes will infest all types of tissues and organs. The size of parasitic nematodes varies considerably from less than 1.0 mm up to several centimetres. Nematodes are not often reported as being a serious problem in farmed fish.
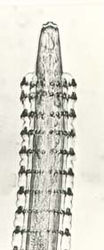
Arthropods
Lernaea (anchor worm)
Lernaea are large (up to 22 mm) crustacean parasites that infest the skin and gills of freshwater fish (Figure 14). Larval and male Lernaea are non-parasitic whereas the female, following fertilisation, will burrow into the skin of the host to become a parasite. The head and mouthparts become modified to form an anchor shape and a small, red pustule forms at the site of penetration (Figure 14a). A large greenish pair of egg sacs develop on the posterior end of the body (Figure 14b), which can be seen protruding from the host. Lernaea have not been reported from Murray cod reared in intensive recirculating aquaculture systems, but are often seen on Murray cod broodfish and other fish grown in outdoor earthen ponds and cages (Rowland and Ingram 1991; Ingram 2004). Light infestations of Lernaea may not be life threatening unless penetration by the parasite is near a vital organ. Heavy infestations may lead to debilitation and infection by bacteria and fungi. Reducing the incidence of Lernaea on farmed fish can be achieved by improving husbandry and management practices in particular, filtration of inlet water to prevent the introduction of free swimming stages of the parasite, and quarantine of all new stock.
Water mites
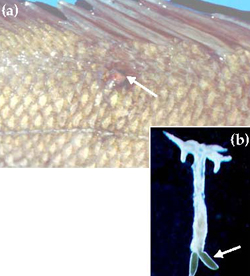
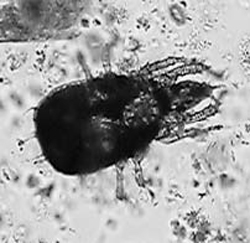
Water mites are spider-like arthropods related to terrestrial mites and ticks. Water mites are characterised by having four pairs of legs, no antennae, and two pairs of appendages on the head called chelicera and pedipalps. Water mites inhabit a wide range of aquatic habitats and are generally not considered as fish parasites. However, under certain environmental conditions they may proliferate and subsequently colonise weak or stressed fish. There has been one report of a water mite species, Histiostoma papillata (Figure 15), attacking and apparently killing juvenile Murray cod held in a recirculating aquaculture system (Halliday and Collins 2002). Fish infested with this mite were listless and had difficulty swimming upright. The fins were damaged and showed signs of haemorrhaging at the bases. Mites were found on the fins, body surface and gills. Large numbers of mites were also found in detritus on the bottom of the tanks and in other parts of the recirculation system. Prevention of water mite infestations should include maintenance of hygienic conditions and water quality and reducing and avoiding stressful conditions.
Ergasilus
Ergasilus, a cyclopoid copepod, is an important ectoparasite having been responsible for massive losses of farmed fish in other parts of the world. Mature females are parasitic whereas immature stages and males are free-living. Ergasilids attach to their host by wrapping their modified second antennae around the filaments of the gills (Figure 16). Attachment and feeding of the parasite causes severe damage to gill tissue. Heavy infestations may result in secondary infections by fungi and bacteria, respiratory difficulties and ultimately death. Although Ergasilus has been reported from Australian native fish (Ingram and Philbey 1999), to date there have been no reported severe outbreaks of this parasite on fish farms in Australia.
Nutritional diseases
Artificial feeds supplied by commercial feed companies contain nutrient and energy sources that are essential for the growth and survival of finfish in aquaculture. Nutritional requirements of fish, especially for protein, amino acids, lipids, minerals and vitamins, varies from one species to another, and for some species the nutritional requirements will also vary according to age. Nutritional deficiencies or imbalances can reduce growth rates and even cause disease in farmed fish. Eleven percent of respondents to a survey of the Murray cod aquaculture industry (Ingram et al. 2004), had experienced nutritional problems.
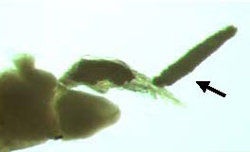
Feed quality
There can be a wide variation in the quality of diets used to feed farmed fish. Key factors that reduce feed quality include poor availability and/or quality of ingredients, poor formulation and processing, a lack of knowledge and understanding of dietary requirements of fish being fed the diet and improper storage of the feeds. Inappropriately stored feeds can become rancid when stored in damp, warm conditions and breakdown of fatty acids in feeds containing insufficient amounts of anti-oxidants can result in the production of harmful toxins. Moulds and fungi growing on the feeds may also produce toxins. Symptoms associated with feeding rancid diets to fish include loss of appetite, darkening of the skin, pallor of gills, swollen, fatty and pale liver and mortality.
Starvation
Starvation may occur as a result of underfeeding (miscalculation of required feed rates or oxygen levels are too low to maintain optimal feeding rates) and/or use of inappropriate feeds (diet composition, digestibility, palatability and size). The most common signs of starvation are loss of condition/weight, reduced activity, increased cannibalism and failure to reproduce. Starvation may occur during the weaning of fingerling Murray cod. Fingerlings should be carefully selected for weaning as stressed fish or fish in poor condition are less likely to accept an artificial diet (Ingram et al. 2001).
Nutritional deficiencies and imbalances
Proteins are long chains of amino acids. There are 10 essential amino acids (EAA) that must be supplied to fish for growth and metabolism. An inappropriate amount of protein and EAA composition in the diet can result in an impairment of protein synthesis in the fish leading to a reduction in protein deposition in skeletal muscle and growth, even if fish are fed to satiation. Other important metabolic processes that amino acids are required for are synthesis of blood proteins (haemoglobin), enzymes and hormones. Some specific diseases that can result from one or more amino acid deficiencies in finfish are skeletal abnormalities (scoliosis/Lordosis), cataracts, fin erosion and "swollen yolk-sac syndrome" (see below).
Essential fatty acids (EFA) include omega-3 and omega-6 fatty acids. EFA deficiencies can retard growth, cause nervous disorders and skin lesions and increased mortality. However, excessive EFA in the diet can cause degeneration of the liver. Feeding high-energy, lipid-rich diets to juvenile and sub-adult Murray cod in captivity over extended periods of time can cause loss of appetite, lethargy, excessive fat deposition in body organs, especially within the body cavity and liver ("fatty liver"), and ultimately death.
Fish have minimum requirements for a number of minerals. Many are absorbed from the water via the gills, but some are obtained through the diet. A phosphorus deficiency will lead to reduced growth and anaemia. Other documented problems include haemoglobin deficiencies and anaemia from a lack of iron and goitre from impaired thyroid metabolism due to an iodine deficiency.
Vitamins are complex organic substances essential to a wide variety of metabolic processes. Deficiencies in vitamins can cause a wide range of conditions including eye disorders and pigment abnormalities (Vitamin A, Vitamin B2), anaemia (Vitamin B12 and folic acid), "brittle" bones, skeletal deformities and poor wound healing (Vitamin C), fatty liver (Vitamin D and choline) and muscular dystrophy (Vitamin E).
Swollen yolk-sac syndrome
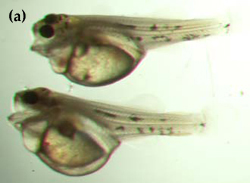
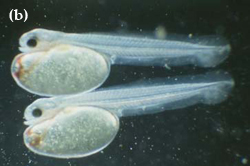
"Swollen yolk-sac syndrome" (SYSS) has caused significant mortalities of the eggs and newly hatched larvae of Murray cod in the hatchery at the Department of Primary Industries, Snobs Creek. Larvae afflicted with SYSS tend to be much paler in coloration than normal larvae, and possess a swollen and misshapen yolk sac as a result of fluid accumulation (Figure 17). Afflicted larvae are lethargic and usually die before feeding commences. Studies have shown that eggs affected by SYSS have significantly lower amounts of essential and non-essential amino acids than did normal eggs (Gunasekera et al. 1998).
SYSS appears to be related to the nutrition of the broodfish held in earthen ponds. Avoiding SYSS may be achieved by ensuring that broodfish obtain a nutritionally balanced diet incorporating ample and varied natural food sources (yabbies and live fish), and that the older broodfish are regularly replaced with younger, new stock.
Long-term management
The incidence of nutritional diseases in farmed fish can be reduced by maintenance of good husbandry and management practices. Use feeds that are specifically formulated for the species and age of the fish. Purchase quality feeds from recognised suppliers. Use appropriate feed rates for the species, age and conditions under which they are being reared. Ensure the palatability, texture and size of feeds, and frequency/timing of feeding are optimised. Feeding strategies may be facilitated by using automatic feeding devices. Feeds should be stored according to the manufacturer's instructions. In temperate areas the storage life of dry compounded feeds may be between 2 and 9 months depending on the quality of the fats used in the feeds, and the conditions under which they are stored. Storage facilities should be kept cool (<20°C) and dry (<75% humidity), and be secured against rodents. Do not use feeds that have a musty or stale smell, are discoloured or appear to be "sweating". Feeds showing any signs of contamination by moulds or fungi should be discarded.
Further information:
General fish disease and nutrition text books. Also see Ferguson 1988; Skrudland 1993; Goddard 1996.
Water quality and environmental factors related to fish diseases
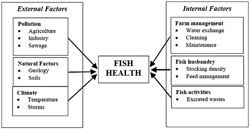
Water is a vital component of any aquaculture venture as it is the medium in which the fish live and grow. Many water quality parameters, if they are altered beyond acceptable limits for the fish being cultured, can influence fish health both directly and indirectly. Each species has a preferred range of water quality parameters and outside of this range will suffer stress, which may lead to disease and even death. There is a high degree of inter-relationship between water quality parameters, which means that a variation in one parameter can influence the toxicity of others.
Fish can be affected by water-borne contaminants from the external environment as well as those arising from their own activities (Figure 18). Potential external sources of water contaminants include pollution from agriculture, sewage or industrial sources as well as natural variations in water quality caused by geology, soils and the climate. Internally, water quality may be influenced by farm management, fish husbandry (including stocking density and feeding) as well as excreted wastes of the fish. The physical action of the fish in stirring up sediments can also have an impact on some parameters. To minimise the risk of water quality-related fish disease the potential hazards of any water supply should always be well understood. Farm management and fish husbandry should also reflect risks associated with the water supply.
In a survey of the Murray cod aquaculture industry (Ingram et al. 2004), 28% of respondents indicated experiencing water quality problems including problems with dissolved oxygen, gas supersaturation, build-up of metabolic wastes (ammonia), and toxic contaminants.
Water quality requirements vary with species, but there is very little data on the tolerance levels of Murray cod or other native finfish. Shepherd and Bromage (1988), Piper et al. (1998), Boyd (1990) and Rowland (1995) provide useful summaries of water quality requirements for various species and forms of aquaculture.
In the absence of specific water quality requirements for Murray cod, Boreham et al. (2004) have attempted to provide a guide to acceptable quality for the intensive production of Murray cod in RAS using historical water quality data from Murray cod aquaculture operations (Table 2). These data were compared against a summary of values published for other species and water quality guidelines for the production of aquatic organisms for human consumption (ANZECC 1999).
Table 2 Acceptable water quality ranges for commercial intensive Murray cod aquaculture (after Boreham et al. 2004)
Parameter |
Suitable concentrations (continuous exposure) (Various sources1) | Recorded from Murray cod aquaculture2 | |
---|---|---|---|
25%-75% | Median | ||
Water temperature (°C) | 5-30 | 20-25 | 24 |
Dissolved oxygen (mg/L) | >5 | 6.9-8.8 | 7.8 |
pH | 5.5-9 | 5.8-6.5 | 6.1 |
Total ammonia (as nitrogen) (TAN) (mg/L) | <3.0 | 0.3-26.5 | 2.9 |
Unionised ammonia (UIA) (mg/L) | <0.02 (pH>8.0) | 0.003-0.02 | 0.008 |
<0.01 (pH<8.0) | |||
Total alkalinity (mg/L) | 20-400 | 8-39 | 15 |
Carbon dioxide (mg/L) | <10 | 20-39 | 31 |
Chlorine (mg/L) | <0.003 | No data | |
Conductivity (us/cm) | <10,000 | 670-960 | 836 |
Total hardness (mg/L) | 20-400 | 205-440 | 273 |
Hydrogen sulphide (mg/L) | <0.002 | No data | |
Nitrate (mg/L) | <100 | 27-77 | 44 |
Nitrite (mg/L) | <0.1 | 0.01-0.63 | 0.24 |
Phosphorus (mg/L) | No data | 0.3-13.0 | 5.1 |
Suspended solids (mg/L) | <25 | 3.1-8.0 | 4.8 |
Dissolved solids (mg/L) | No data | 171-424 | 244 |
Turbidity (FAU) | <25 | 3-10 | 5 |
BOD (5 day) (mg/L) | <15 | 2-23 | 13 |
COD (mg/L) | <15 | No data | |
Metals | |||
Aluminium (mg/L) | <0.03 (pH>6.5) | No data | |
<0.01 (pH <6.5) | |||
Cadmium (mg/L) | <0.003 | No data | |
Calcium (mg/L) | 10-160 | No data | |
Copper (mg/L) | <0.005 | No data | |
Iron (mg/L) | <0.05 | No data | |
Lead (mg/L) | <0.01 | No data | |
Magnesium (mg/L) | <15 | No data | |
Manganese (mg/L) | <0.01 | No data | |
Mercury (mg/L) | <0.001 | No data | |
Zinc (mg/L) | <0.01 | No data |
- Shepherd and Bromage (1988), Boyd (1990), Rowland (1995), Piper et al. (1998) and ANZECC (1999),
- Summary of data collected during the the project entitled Development of Intensive Commercial Aquaculture Production Technology for Murray Cod (FRDC Project No. 1999/328) (Ingram and De Silva 2004).
Water quality parameters discussed here are relevant to all species and the unacceptable levels indicated should also be used as a guide for Australian native fish species. Water quality parameters that most commonly cause fish health problems in Australia include:
- Dissolved gases - particularly dissolved oxygen. Other gases which may be important include: carbon dioxide; chlorine; hydrogen sulphide; methane and nitrogen.
- Metabolic waste products - particularly ammonia and nitrite.
- Other important parameters - pH, temperature, heavy metals, water hardness and salinity.
- Water-borne contaminants and other problems: toxic organic compounds, biocides, noxious algae, weather, predators and pests.
There are several key water quality parameters that should be tested for when any fish disease is suspected, especially temperature, dissolved oxygen, pH, ammonia and nitrite. Temperature and dissolved oxygen measurements should be taken in situ at the time of water sampling. The farmer should ensure that meters are kept in good repair and regularly calibrated to ensure accurate data. Other key parameters can be measured using commercially available water quality test kits or strips. If the key parameters are within acceptable ranges, it may be necessary to submit a water sample to a commercial laboratory for further analysis
Dissolved gases
Dissolved Oxygen
Environmental hypoxia is a low concentration of dissolved oxygen (DO) in the water and is perhaps the greatest risk in aquaculture. The sensitivity of fish to low concentrations of DO differs between species, life stages and life processes (feeding, growth, reproduction etc.), although a minimum level of 5 mg/L is considered ideal for optimal growth and reproduction. Below this level food consumption decreases and growth slows. A DO of less than 2 mg/L is very stressful and may lead to opportunistic infections (bacteria, fungi, parasites). DO concentrations of less than 2 mg/L have been recorded in fry rearing ponds used to rear Murray cod fingerlings (Ingram 2001). Problems with hypoxia in intensive Murray cod aquaculture facilities are being avoided by supersaturation of inlet water with pure oxygen (Boreham et al. 2004). Low DO levels may be caused by:
- Excessive plant respiration. Although plants (inc. algae) produce oxygen during daylight hours, during darkness they consume oxygen. In ponds where algal growth is very high, DO levels may be depleted during the night, and DO levels may also become depleted after several overcast days.
- Increasing water temperature, which decreases the solubility of oxygen in water.
- Presence of decaying organic matter. Contamination of the water source with sewage, animal manures or silage, algal die-off in ponds or accumulation of waste feed and faecal particles in the farming system.
- Use of groundwaters that often have low DO levels and with high iron contents
- Use of industrial wastewaters containing chemicals that will reduce DO levels.
- Overstocking and overfeeding resulting in low DO levels.
- Breakdown of the aeration systems.
Symptoms:
Fish cease feeding and may "gasp" at the water surface or gather at water inlets. Death occurs with opercula flared and mouth agape. Often large fish die and small fish may survive. The symptoms of hypoxia can also be symptomatic of gill damage (which impairs oxygen uptake) and anaemia, which reduces oxygen bioavailability.
Diagnosis:
Direct measurement of DO and temperature with meters.
Immediate action:
Increase aeration, increase water exchange, stop feeding, reduce stocking density. Monitor ammonia and nitrite to ensure that biological filtration is functioning in RAS.
Long-term management:
Routine (daily) monitoring of DO in ponds (at dawn) and tanks. In ponds, provide supplementary aeration that is automatically timed to operate during periods of low DO (especially early morning). Install and maintain emergency back-up aeration and oxygenation systems. Do not overstock tanks and ponds, improve feed management and/or increase water exchange.
Gas Supersaturation - Gas Bubble Disease
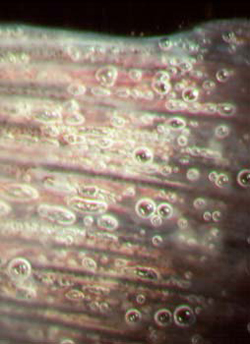
Gas supersaturation may be caused by excessive plant photosynthesis during daylight hours in ponds with dense algal blooms, inflow water saturated with gases as a result of heating under pressure, water inflow pipes sucking in air (prior to pumps) and groundwaters that are supersaturated with nitrogen and/or carbon dioxide. Most gas emboli are produced by excess nitrogen. Oxygen rarely causes gas bubble disease because it is assimilated metabolically and thus less likely to form persistent bubbles.
Symptoms:
Fish become listless and float to the surface. Small bubbles can be seen forming in superficial blood vessels typically on the gills and fins (Figure 19) and behind the eyes.
Diagnosis:
Measure water with a saturometer (an oxygen meter will give an indication of oxygen supersaturation only). Small bubbles will immediately form on any object placed in the water.
Immediate action:
Eliminate excess gases from the water source by aerating water in a reservoir to allow gases to equilibrate.
Long-term management:
Install de-gassing devices on pipelines and systems before the water enters the culture vessels.
Carbon dioxide
Carbon dioxide (CO2) is essential for plant growth and is usually present as a free gas, or bound with other elements to form bicarbonates, carbonates and organic compounds. High levels of free CO2 can cause problems at low pH levels (pH<7.0). The main sources of CO2 are: respiratory wastes of the fish; decomposition of organic matter; respiration by aerobic bacteria (such as within biological filters); groundwater; and the atmosphere. High levels of free carbon dioxide (CO2) have caused problems in RAS growing Murray cod. Boreham et al. (2004) reported CO2 concentrations of between 20-39 mg/L in a RAS growing Murray cod, which were considerably higher than the recommended max concentration of 15 mg/L for continuous exposure (Table 2). These results highlight the importance of degassing of CO2 in RAS. High levels of CO2 interfere with oxygen uptake. Symptoms of fish exposed to excess CO2 include dyspnoea and chronic inflammation and calcium carbonate disposition in the kidneys and epaxial muscles. If CO2 poisoning is suspected (ie >12 mg/L CO2), and all other possible causative agents are ruled out, immediate action should include increasing aeration, reducing stocking density, and in ponds, addition of calcium hydroxide (Boyd 1990). Long term management includes use of mechanical aeration and oxygenation systems as well as use of degassing devices to strip CO2. Trickle biological filters in RAS should be well aerated to facilitate stripping of CO2 from the water.
Chlorine/chloramine poisoning
Chlorine is commonly used to treat town water to make it suitable for human consumption. Ammonia is sometimes also added to stabilise the chlorine, and reacts producing chloramines. Both chlorine and chloramine are extremely toxic to fish and can cause acute or sub-acute toxicity. Symptoms of chlorine/chloramine poisoning include breathing difficulty, dyspnoea and death. Gill tissue of affected fish becomes necrotic. Fish exposed to chlorine poisoning appear to have improved survival if the water is super-saturated with oxygen for several days and the temperature is lowered. A reliable water quality spectrophotometer-based test kit can measure chlorine. Chlorine can also be detected by smell. Any detectable amount of chlorine or chloramine is undesirable. Chlorine can be removed from water by vigorous aeration, but chloramines are not so readily removed. Water may also be filtered through activated carbon or treated with a chemical neutraliser such as sodium thiosulphate.
Hydrogen sulfide poisoning
Hydrogen sulfide (H2S) forms from the reduction of sulphate under anaerobic conditions. It is more of a problem in brackish water/marine systems, but can occur in freshwater systems where there is an accumulation of organic matter. Disturbing pond sediments can also release this gas from the mud. H2S interferes with respiration causing hypoxia. Concentrations of H2S between 0.5-1.0 mg/L can cause acute mortality. Presence of H2S is often detected by a characteristic "rotten egg" smell, and can be measured by a reliable water quality spectrophotometer. H2S can be removed from water by vigorous aeration or use of a degassing device. Raising pH and lowering temperature also reduce toxicity. Build-up of H2S in ponds can be avoided by maintaining aerobic conditions with supplementary aeration. Regular addition of fresh water also assists in reducing H2S concentrations.
Metabolic Waste Products
The waste products from the fish themselves, particularly nitrogenous wastes, can adversely affect water quality if they are allowed to accumulate. Nitrogen is administered to the fish in the form of protein in food and is excreted as ammonia, primarily across the gills. Decaying organic matter within the culture system, such as waste food, faeces, dead plants or animals also produces ammonia. Under aerobic conditions, ammonia is oxidised by bacteria to nitrite and then to nitrate (Figure 20). Nitrate is generally considered non-toxic to fish, but both ammonia and nitrite are toxic under certain conditions.
Ammonia poisoning
Ammonia poisoning is one of the most common water quality problems in aquaculture. It is the primary nitrogenous waste product of fish, but also comes from the decay of organic matter such as waste feed and algae. Ammonia can cause acute mortality, but most often it presents as a sub-lethal stress. Potential causes of elevated ammonia levels include overcrowding of fish, recent medication or other chemicals added, newly established recirculation systems, failure of biological filters, reduced water flow and accumulation of waste feed or other organic matter. Ammonia is present in water in two forms unionised ammonia (NH3), which is toxic to fish, and ionised ammonia (NH4+), which is much less toxic. The proportion of unionised ammonia increases as pH and temperature increase (Emerson et al. 1975). Appendix 4 provides a table for calculating unionised ammonia at different pH's and temperatures. Approximate lethal and sub-lethal levels for unionised ammonia are >1.0 mg/L and >0.05 mg/L, respectively. Concentrations of unionised ammonia recorded from Murray cod aquaculture operations to date have generally been less than 0.02 mg/L (Table 2) which is acceptable for continuous exposure. Ammonia toxicity also varies with salinity, water hardness and other stressors.
Symptoms:
Acute ammonia toxicity can cause behavioural abnormalities such as hyper-excitability and fish often stop feeding. Sub-lethal ammonia poisoning decreases growth and disease resistance.
Diagnosis:
Chemical measurement of total ammonia, pH and temperature using appropriate test kits and meters. Use attached tables to convert total ammonia to unionised ammonia (NH3) (Appendix 4).
Immediate action:
Dilution by addition of fresh water, stop feeding, decrease stocking density, reduce temperature and reduce pH. Some types of Zeolite may also be used to strip ammonia from water.
Long-term management:
Improve husbandry practices and take an active approach to managing the health of stock. Monitor ammonia concentrations (daily in intensive recirculating systems, less frequently in ponds), maintain hygienic conditions, avoid waste accumulation in culture system, improve water quality, ensure sufficient volume of biofiltration media in recirculation systems, manage stocking densities, feeding rates and water flows.
Nitrite poisoning (Brown blood disease)
Nitrite is an intermediary product in the oxidation of ammonia to nitrate by bacteria (Figure 20). Many of the circumstances that lead to a build up of ammonia can also lead to nitrite poisoning. Peaks of ammonia and nitrite occur in the weeks following the commissioning of newly established biological filters. In ponds, nitrite peaks can occur in autumn as the temperature optima of the two bacteria (Nitrosomonas and Nitrobacter) are different leading to nitrite accumulation. Susceptibility to nitrite toxicity varies enormously between species. There is no data on the toxicity of nitrite to Australian native fish. Concentrations of nitrite less than 0.1 mg/L are considered suitable for continuous exposure, however, higher concentrations in excess of 0.6 mg/L have been recorded from some Murray cod aquaculture operations (Table 2).
Symptoms:
Behavioural changes are similar to characteristics of hypoxia. In acute to chronic cases, dyspnoea may occur and gills become light tan to brown in colour due to a change in blood colouration.
Diagnosis:
Test for nitrite using a chemical test kit.
Immediate action:
Dilution by addition of free water. Nitrite toxicity is reduced by addition of salt (<50 mg/L salt is usually sufficient) as the chloride ion competitively inhibits nitrite uptake across the gills.
Long-term management:
As for Ammonia poisoning.
Other important water quality parameters
Temperature
Temperature dramatically affects fish metabolism and each species has a preferred temperature range. Absolute temperature ranges do not exist because tolerance depends on several factors, including the acclamation history, salinity, life-stage and reproductive status. All fish are susceptible to rapid changes in temperature, but seem to tolerate a rapid decrease in temperature better than the reverse. At the extremes, fish may be stressed to the point where growth and survival are affected. At low temperatures, outside the fish's optimal range, the immune system is suppressed, which increases susceptibility to other fish diseases. Fish stocked outside their natural range may exhibit "winter kill". Temperature stress depends not only on how low or high the temperature becomes, but how quickly it arrives at the temperature. Water temperature is readily measured by thermometer or meter.
Under ambient conditions within the natural range in the Murray-Darling Basin, Murray cod may be exposed to temperatures from less than 10°C to in excess of 30°C. Not surprising, studies have shown that the growth of Murray cod is affected by water temperature. At temperatures below 16°C, feeding is reduced and growth is negligible (Ingram 2004). However, at higher temperatures, fish may stop feeding and become stressed, predisposing them to disease. Ingram (2004) attributed mortalities in Murray cod during a cage culture experiment, to stress and other factors associated with water temperatures that reached 30°C. The optimal water temperature for the culture of Murray cod is not clearly defined, however, under current industry practices Murray cod are being reared at temperatures between 20°C and 26°C in intensive RAS.
pH
A pH range of 6.5-9.0 is generally recommended for freshwater fish. Outside of this range is stressful to fish and levels less than 4.0 (acidic) and greater than 11.0 (alkaline) are usually lethal. Fish acclimatised to low or high pH levels, or fish used to pH fluctuations are more tolerant of pH changes than fish kept under more stable conditions. pH can affect the toxicity of other chemical parameters. Low pH levels increases the toxicity of aluminium to fish whereas high pH levels increase the toxicity of ammonia. Sources of low pH water include groundwater in contact with silicate minerals, and waters draining from or overlaying acid sulphate soils. The metabolic activity of fish and other aquatic organisms produces acid, which can gradually lower the pH in some systems. Some groundwaters may also contain high pH levels. Both meters and test strips are used to measure pH levels.
Exposure to highly acidic waters increases mucus production of the gills causing respiratory difficulty and mortality in extreme cases. Exposure to highly alkaline waters can cause cloudiness of skin and gills, and mortality in extreme cases. Extreme pH levels can be treated by dilution with fresh water and/or the addition of a buffering agent to lower/increase pH. Monitor pH levels regularly (daily in intensive recirculating systems, less frequently in ponds) and avoid build-up of large algal blooms in ponds.
Murray cod cultured in RAS are often being exposed to pH's between 5.8 and 6.5 (Table 2), whereas in fertilised earthen fry rearing ponds, juveniles are exposed to pH's between 5.6 and 10.4 (Ingram 2001). The high pH readings reported in fry rearing ponds are the result of dense algal blooms removing CO2 by photosynthesis (Boyd 1990). In contrast, in RAS there is a tendency for the pH to decrease as a result of respiration of fish and an associated build-up of CO2 (Grace and Piedrahita 1994). However, operators of RAS often actively manage pH in RAS systems by stripping CO2 and addition of a buffering agent such as sodium bicarbonate or sodium hydroxide (Bisogni and Timmons 1994; Grace and Piedrahita 1994). Managing pH is a balancing act. If pH is allowed to become too acidic, fish may become stressed and nitrification processes are impaired, whereas increasing pH will increase the proportion of ammonia that is toxic to fish.
Salinity
Salinity is a measure of all ions in water and is most commonly expressed as parts per thousand (ppt). Freshwater generally has less than 0.5 ppt salinity while seawater is 30-40 ppt. Salinity tolerance of fish varies with species and length of exposure.
Heavy metals
Fish are sensitive to dissolved metals and metals are most toxic in low alkalinity waters in which high concentrations of metals remain dissolved. Metal contamination may occur from the following sources:
- Leaching from lead, copper or galvanised (containing zinc) plumbing fittings.
- Groundwater (especially soft, acid water) that may have high concentrations of metals.
- Rainfall run-off from soils that are poorly buffered or are contaminated (e.g. mine wastes).
Symptoms of metal poisoning vary and depend on the metal and fish species (see Sorensen 1991). Effects can be chronic or sub-lethal leading to a gradual decline in fish health and reduced immunity to other diseases. Most heavy metals primarily affect the gills. Standard water quality test kits used in aquaculture are usually not sensitive enough to detect metal concentrations that affect fish. Water samples must be submitted to a laboratory specialising in water analysis. Submission of a fish samples for examination may also assist diagnosis. Eliminating metal fittings (especially those containing, lead, copper or zinc) from aquaculture systems reduces the risk of metal poisoning.
Water-borne contaminants and other problems
Organic compounds such as PCBs, detergents and hydrocarbons, pesticides, herbicides and molluscicides can be extremely toxic to fish and may reach aquaculture water supplies by accidental spillage or contamination and runoff from agricultural and industrial lands. Clinical symptoms vary with the type of compound but tend to include distress, respiratory failure, avoidance behaviour and death.
Avoiding water quality problems
Aquaculture requires good water quality and prior to development of an aquaculture operation, the water source should be thoroughly checked to ensure that it is free from contaminants including organic, agricultural and/or industrial pollution, heavy metals, excessive suspended particles and pathogens or other undesirable aquatic organisms.
Some water supplies have characteristics that may make them more or less suitable for an aquaculture venture. Groundwater tends to have a more constant temperature than surface waters, but often has low levels of dissolved oxygen and may have elevated levels of metals, particularly iron. The mineral balance of groundwaters may not, in some circumstances, be ideal for finfish, but very little work has been done in this area and there are no established guidelines by which to assess the suitability of groundwater sources for specific species. Surface waters are subject to greater variations in temperature and are more vulnerable to pollution or external contamination. Town water supplies have a fairly constant temperature and quality, but are usually dosed with chlorine and/or chloramines which is extremely toxic to fish.
ANZECC Water Quality Guidelines provide recommended water quality standards for the protection of cultured fish, molluscs and crustaceans (ANZECC 2000) (see Appendix 5). If the water supply does not meet these criteria, some form of pre-treatment may be required. Information on water quality in major rivers, streams and groundwaters can be obtained from state Environmental Protection Agencies as well as regional/Local government Water Authorities, Catchment Management Authorities and Irrigation Authorities. These organisations can also provide information on potential sources of pollution.
Submitting water samples for analysis
Specific recommendations for sample collection vary with the type of substance being measured and with how quickly the sample can be submitted for analysis. The laboratory should be contacted prior to submitting the samples as many have specific requirements on the type of container to be used (some require that you use their bottles) and methods of preservation. Ideally, a clean polyethylene bottle should be used to collect the sample (except if pesticide contamination is suspected when glass bottles are best). In urgent situations, a still mineral water bottle may be used (if this is acceptable to the laboratory). The water bottle should be rinsed at least three times with the water to be sampled before filling to the top and sealing with a lid. The sample should then be chilled, frozen or preserved on the advice of the laboratory, and submitted for analysis as soon as possible.
Other fish health issues
Stress and Fish health
Stress is considered as an environmental externality that reduces the ability or capacity of a fish to maintain health and well-being. More importantly, stress can reduce growth and illicit poor fish health. Stressed fish suffer from depressed immune systems and consequently lowered resistance to disease or parasite infestation.
Stressors in fish farming include:
- Handling. Capture, netting, grading weighing, tagging, injecting.
- Transport.
- Territorial behaviour. Aggression by dominant fish, competition for food, competition for refuges, competition for mates, etc.
- Predation pressure.
- Confinement during culture, handling or transfers. Confinement associated with overstocking.
- Chemicals and water quality.
- Temperature changes.
- Parasitism/disease.
Some factors may not be stressful by themselves but combinations of several of these factors, acting in synergy, can have a cumulative affect on inducing stress. Therefore, care should be taken to minimise the number of stessors acting upon fish at any one time, such as handling fish when ammonia concentrations are excessively high.
Stages & sites infected:
Juveniles, sub-adults and adults.
Symptoms:
Loss of appetite. Fish that have been poorly handled, damaged during transport, or injured by territorial behaviour, can often be recognised by having severely frayed fins, lost scales, marks or abrasions on the body and cloudy eyes.
Immediate action:
Reduce/eliminate stressors.
Long-term management:
Improve husbandry practices and take an active approach to managing the health of stock. Reduce excessive handling of fish. Where possible, when handling and transporting fish, use light dosages of anaesthetics and salt to reduce stress. Not only does salt help to reduce stress, but also promotes the production of mucus and healing, is beneficial to osmoregulation and has anti bacterial, anti-fungal and anti parasitic action. Routinely grade fish to reduce size variation. Maintain hygienic conditions and water quality. Manage stocking densities, feeding rates and water flows.
Further information:
Iwama et al. 1997.
Aggression and Cannibalism
Murray cod are known to be aggressive, territorial and predatory in nature, and it is not surprising that they are also capable of attacking and consuming their own kind. Cannibalism appears to be prevalent in culture tanks where densities are low and/or the size range of fish is great. Other factors have also been shown to affect the rate and extent of cannibalism, including availability and nutritional composition of food, feeding frequency, water clarity, light intensity and the presence of refuges. Increased turbidity may reduce territorial and aggressive behaviour and cannibalism. Murray cod are less likely to attack and consume siblings that are already dead. Cannibalism can lead to shifts in the size variation of stock and increase the risk of disease. Stress associated with cannibalism pressure may reduce growth rates in smaller fish and lead to increased susceptibility to disease. Larger fish may also suffocate while attempting to ingest smaller fish (Figure 21).
Stages & sites infected:
Juveniles, sub-adults and adults.
Symptoms:
Damage to dorsal, anal and caudal fins, loss of scales or physical marks on the sides of the body, unexplained loss of fish. The presence of large fish with grossly expanded abdomens or with tails protruding from the mouth.
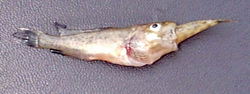
Immediate action:
Grade fish to reduce size variation and maintain densities to reduce stress associated with undercrowding. Increasing stocking densities may reduce the ability of fish to establish territories and thereby reduce the impacts of territoriality and aggression.
Long-term management:
Improve husbandry practices and take an active approach to managing the health of stock. Ensure that fish are receiving ample feed to sate appetite. Feeds should be specifically formulated and appropriately sized for the species, and feeding frequency/timing optimised to correspond with peak feeding periods. Feeding strategies may be facilitated by using automatic feeding devices. Where possible distribute feed over a large area or have several feeding stations. Remove obvious cannibals and dominant individuals. Maintain optimal stocking densities. Grade fish regularly to reduce size variation. Juveniles may require grading every 3-4 weeks, but as fish grow the need to grade will reduce.
Further information:
Smith and Reay 1991; Hecht and Pienaar 1993.
Predation
Aquatic insects
Many species of aquatic insects are predatory, particularly species of the Aeshnidae (Odonata), Dytiscidae (Coleoptera) and Notonectidae (Hemiptera). In fry rearing ponds, aquatic insects, particularly when abundant, can compete with fish for food, such as zooplankton, or even prey directly on fish larvae and small fry. Certain species of dragonfly larvae are major predators of small fish in aquaculture ponds and when abundant can be a major threat to the survival of larvae and fry. Removing marginal vegetation from the perimeter of fry ponds prevents some dragonfly species from colonising the pond by eliminating egg deposition sites used by the adults. Most aquatic hemipterans (bugs) are carnivores, feeding by piercing the body of their prey with their mouth-parts and sucking out the body fluids. Larger species especially the Belostomatidae, Nepidae and Notonectidae, can prey on small fish. Studies at the Narrandera Fisheries Centre have shown that large species of notonectids will prey on the fry of golden perch and silver perch (S. Thurstan, pers comm.). However, if there is an abundance of food in the ponds, predation by notonectids is reduced (S. Thurstan, pers. comm.). Some beetle species have predatory larvae, especially the dytiscids, which are notorious for their ability to attack and eat small fish. However, there are few records, none convincing, of adult dytiscids attacking fish.
Vertebrates
Turtles are mostly carnivorous, feeding on fish, tadpoles, molluscs and crustaceans, and so are a nuisance in aquaculture ponds. Since all native reptiles, including turtles, are protected by legislation, offending animals should be relocated rather than destroyed.
Birds that most frequently occur around aquaculture ponds are the wading birds (herons and egrets), diving birds (cormorants and darters) and web-footed birds (ducks, grebes etc.). Some of these species are considered a pest in aquaculture ponds. The cormorants are notorious fish predators and can eat up to 27% of their body weight per day, with daily intake ranging from 125g/day for little pied cormorants to in excess of 500g/day for black cormorants (Barlow 1995). Consequently, the impact of fishing by flocks of cormorants on fish in aquaculture ponds can be quite devastating. Apart from devouring small fish, cormorants also cause injury and stress fish. Wading birds may take their toll on fish in ponds, but their impact is limited to wading-depth water around the edges of ponds. Controlling bird predation can be costly and time consuming. The use of harassment devices such as noise makers and scarecrows to control bird predation are mostly ineffective because the birds eventually become accustomed to them. More often than not, lethal control measures, namely the use of fire-arms, are used. Although all native birds, including cormorants, are protected by legislation, a permit can be obtained to control pest species. However, the most effective method, though expensive, is to exclude the birds by enclosing ponds in bird-proof netting.
The native water-rat (Hydromys chrysogaster) has amphibious habits and, although preferring to live near permanent water bodies such as rivers and streams, it is occasionally found around aquaculture facilities. Water rats have broad, partially-webbed hind feet, water-repellent fur and a thick, white-tipped tail. Water rats are active carnivores and will prey on fish in aquaculture facilities. The water-rat is protected by legislation, so the preferred method of control for this predator is to trap them alive and remove them to a new location.
Further information:
Ingram et al. 1997; Ingram 1997.
Eye disorders
Cultured fish occasionally develop eye disorders, the more common of these are exophthalmos (popeye), lenticulitis (cataracts) and keratitis (ketatopathy). Fish with exophthalmos typically have swollen or protruding eyes and occasionally there may be gas bubbles present within the eye. Lenticulitis affects the lens of the eye, which becomes cloudy or opaque. In fish suffering keratitis the cornea of the eye is injured resulting in a dulling, cloudiness or discolouration of the cornea, inflammation and/or ulceration.
The causes of eye disorders are varied and include nutritional deficiencies or imbalances (lenticulitis and keratitis), parasitism (viral, bacterial, fungal, protozoan and metazoan), poor water quality, gas supersaturation, presence of toxicants, physical trauma and stress associated with captivity (especially keratitis), osmotic imbalances and excess sunlight. Indeed the presence of some eye disorders may serve as an indicator of unfavourable culture conditions. Some disorders, especially if left untreated, can lead to permanent loss of sight and even death. Eye disorders can severely impact on aquaculture operations. Some fish may be lost due to death while blindness in other fish may result in reduced feeding and growth. Customers may also reduce sales of fish as a result of devaluation or rejection.
Long-term management:
Improve husbandry practices and take an active approach to managing the health of stock. Maintain hygienic conditions, avoid poor water quality, reduce/avoid stressful episodes, control other diseases and avoid overcrowding and excess handling. Use nets with soft netting, hold fish in smooth-walled containers and ensure nutritionally appropriate diet is used.
Further information:
General fish disease text books. Also see : Hargis 1991.
Chronic erosive dermatopathy
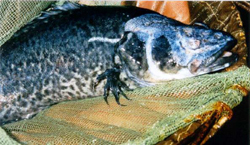
Chronic Erosive Dermatopathy (CED) was first identified as a problem on Murray cod in intensive aquaculture operations in the mid- to late- 1990's. CED is an ulcerative condition that affects the sensory pores and tissue overlaying the lateral line (Figure 22). In extreme cases, the eyes and fins are also affected. Few mortalities appear to occur and fish continue to grow. The causative agent of CED has yet to be conclusively identified. Viruses, protozoan and metazoan parasites are not implicated. Bacterial pathogens are present but considered to be secondary/ or opportunistic colonisers of infected areas. Poor nutrition is not considered a key factor. Currently the causative agent/agents of CED is thought to be a waterborne toxicant. All farms that have experienced CED were using bore water. Elevated and/or imbalances of certain elements and/or compounds in the bore water, perhaps operating in synergy, may be directly affecting the sensor nervous tissues over the head and body of the fish. Interestingly, afflicted fish have been able to regenerate eroded tissues within 12 months after being placed in a surface water source.
Immediate Action:
Replace bore water with surface water of appropriate quality for the species.
Further information:
Trott 1999; Humphrey et al. 2000; Trott 2000; Baily 2003, Baily 2003
Fish health management
The principal objective of fish health management strategies in intensive aquaculture operations is to maintain the health and wellbeing of stock, while optimising fish production. Key actions, which are critical to achieving this objective are:
- Take an active approach to managing the health of stock
- Maintain hygienic conditions
- Sterilise inlet water
- Guard against poor water quality
- Monitor water quality regularly
- Seek health certification for new stock
- Quarantine all new stock
- Minimise unnecessary stress
- Monitor health of stock regularly
- Feed fish an appropriate diet (composition, size, amount etc.).
Water supply treatment
A major source of pathogens to intensive aquaculture facilities is the water supply. Both the quantity and quality of water used in aquaculture are critical to the well-being of fish under culture. A regular and abundant water supply is essential for any aquaculture operations. A number of water sources are currently being used for Murray cod farming including, surface waters (streams, rivers, lakes and dams), groundwaters (bore water) and domestic/urban waters (Ingram and Larkin 2000). Ideally these waters should be regular and reliable, free of pathogens, and free of pollutants (organic, industrial and urban) and consequently, regardless of the water source, some form of pretreatment will be required before it is used in the culture system.
Untreated surface waters pose the greatest risk of pathogen contamination, especially if fish are present in the water supply. Treatment options may include combinations of the following:
- Mechanical screening (sand filters, carbon filters, screen filters) to remove particulate matter.
- Chemical treatment (chlorination followed by dechlorination and/or aging) to eliminate pathogens.
- Ozonisation to eliminate pathogens
- UV sterilisation to eliminate pathogens.
- Elimination of fish from the water source (where possible).
Bore water is generally considered to be pathogen-free, however, since water quality varies widely from one source to another, groundwater requires thorough testing before use in aquaculture. Concentrations of some parameters (eg. iron, hardness, conductivity and dissolved oxygen) may render the water unsuitable for fish culture. Some form of pretreatment may be required before it is suitable for fish.
Domestic waters provide a clean, hygienic alternative water source. However, the presence of high levels of chlorine compounds (used by councils to disinfect water for human consumption) necessitates the need to "age" or dechlorinate the water before use.
Managing new stock
Another important source of pathogens is new fish stock. To ensure that diseases are not introduced though restocking of aquaculture production facilities, all new stock, regardless of the source, must be quarantined. All aquaculture facilities, regardless of size, must have a quarantine facility that is separate from the rest of the facility. The quarantine system should be physically isolated from the other facilities (separate room or building). Water used in the quarantine system must be totally separated and must not be allowed to mix with the water of other culture systems within the facility. Equipment used in the quarantine area should not be used in other areas of the facility. Minimise traffic through the quarantine area and disinfectant footbaths should also be used at the entry/exist of the quarantine area. New stock should be quarantined for at least 2 weeks before introducing to the production facility. During that time, fish should be checked regularly for signs of disease, and should be given prophylactic treatments, such as salt and formalin baths, to eliminate external parasites which have previously been identified as a major health problem for farmed Murray cod. Finally, the water quality conditions within the quarantine system may be different to that within the production system. Therefore, gradually acclimatise new stock to production system water conditions during the quarantine period.
New stock should appear healthy at the time of delivery. The presence of fish that appear weak, underfed or unhealthy is a clear sign that health problems may arise. Always purchase new stock from a reliable supplier, and always seek health certification. As an added measure of security, samples of fish should also be sent to a fish health specialist for disease screening.
Hygiene
A comprehensive cleaning and sanitising program should be established to maintain hygienic conditions within the facility. This program should include the treatment of all equipment that may be exposed to pathogens, including tanks, nets, buckets, meters, and floors. Sharing of equipment between different systems of the facility should be prohibited. Uneaten and spilled food and sick or dead fish should be immediately removed from culture systems. Cleaning of the floors to prevent the build-up of debris, including spilt feed, must be a regular activity. There are numerous cleaning and sanitising chemical compounds and agents. However, there is limited information on the performance/efficacy of these products, which makes it difficult to establish a quality control program (Flick 1998). Care should be taken in the use of these products, as they may be hazardous to humans, fish and the beneficial microorganisms within the systems (especially the biological filter). Some commonly used sanitisers include chlorine solutions, iodine solutions, quaternary ammonium solutions, ultraviolet radiation, chlorine dioxide, phenols, alcohols and aldehydes (eg. formaldehyde) (Flick 1998).
Hygiene management should also extend to staff. Management practices may include provision of anti-bacterial soaps for washing hands, use of footbaths and disinfection of footwear and restricted visitation of people from other fish farms.
Humane slaughter and destruction of stock
Coinciding with growing public concerns about the slaughter of finfish, the Victorian Prevention of Cruelty to Animals Act (1986) was amended in 1995 to include the humane killing of fish and crustaceans. On an international scale, the subject of humane slaughter of finfish has been researched extensively. One report reviews a project carried out in the UK that brought together animal welfare groups, retailers, research groups and aquaculture stakeholders to investigate the issue (Robb 2000). It is important to note that the type of slaughter method and degree of stress on the fish during harvest can have a significant effect on product quality.
The following ten slaughter methods were each ranked with respect to welfare and quality: death in air; death on ice; carbon dioxide; carbon dioxide & ice; AQUI-S & ike jime; percussive stun; ike jime; head only electric stun; whole body stun (short); and whole body stun (long). Measured welfare found that none of the most popular methods, death in air, death on ice or carbon dioxide, resulted in humane slaughter. In order to achieve effective welfare it was determined that either a rapid kill was required or stress minimised if the kill was not immediate. The four possible options that achieved humane slaughter were percussive stunning, ike jime (brain spiking), AQUI-S and electrical stunning.
Percussive stunning is inflicting a fast, firm blow to the head, using a club such as a wooden dowel or a mechanical bolt gun. While being a very effective method of humane killing of large fish, the quality of the final product will depend on operator experience and fatigue. Ike jime is a technique that sees a sharp spike inserted into the brain, that interrupts nervous activity, reduces muscle twitching and slows rigour mortis, giving rise to higher quality flesh. This method is effective only when under-taken by experienced operators and is more suited to larger species. AQUI-S is a type of eugenol anaesthetic that is produced from clove oil. Using AQUI-S with aerated or oxygenated water at the prescribed dose rate results in the fish becoming heavily sedated. The fish can then be killed quickly during processing. One advantage of this technique is that it allows specific sized fish to be targeted and smaller ones can be graded out and returned to recover in a tank or pond. Care must be taken not to under or overdose the stock. In early trials, electrical stunning resulted in broken vertebrae and haemorrhaging in many fish.
Subsequent experiments varying the frequency and current duration overcame these problems.
Whatever the type of humane slaughter technique adopted by the farmer, there will be costs incurred. The technique used must meet humane standards, but it must also be practical and economically viable. Not all of the mentioned techniques are practical for handling large volumes of relatively small fish, which is a situation at many aquaculture operations. In order to address the issue of animal welfare during slaughter different aquaculture sectors are starting to incorporate humane slaughter techniques into their industry Codes of Practice.
Disposal of mortalities
Fish carcasses may represent a potential source of certain fish pathogens, especially viruses and bacteria. Fish mortalities occur during production, and regardless of the reasons for the mortality, carcasses require prompt removal from culture units and careful, effective disposal. Transportation of mortalities from site generates potential risks of infection spread, and should only be undertaken with direction from appropriate authorities, if no on-site alternative can be found. Current practice involving pit disposal on site is poorly defined, with no direction on position, depth construction or limitations on materials to be placed there. Pit disposal, if not carefully administered, may represent a potential source of reinfection for stock.
If pit disposal is to be used, the pit must be in a clearly marked area, and be lined with a non-permeable barrier to prevent leakage to the surrounding soil and groundwater table. Recommended linings include 2-5 mm high density polyethylene (HDPE) or clay compacted to a standard of 1x10-9 m/s with a minimum thickness of 0.3 m. Pits should be situated at least 100 m away from surface waters (waterways, drainage lines etc.) and a minimum watertable depth of 2.0 m. Lime should be added to the carcasses being disposed of and final burial should involve a top dressing of soil placed over the carcasses.
Dealing with fish health issues
Identifying the problem
Monitoring is an important part of early identification, management of disease problems. A decision support flowchart has been prepared to assist farmers in the identification of common health problems in intensive aquaculture systems (Figure 23).
Changes in behaviour may be the first sign that fish are being stressed. These changes may include:
Reduced or cessation of feeding, lethargy, floating near the water surface and/or swimming with the head upwards, "flashing" or erratic swimming, and increased or laboured respiration (as indicated by "gasping", flaring of opercula or rapid opercula movement). Physical chances may become apparent, such as abnormal growths, lesions or discolouration on the body surface, loss of some scales and cloudiness of eyes. Fins may become tattered or eroded. The gills may become clogged with mucus and the filaments may appear swollen or fused together.
Water quality should always be checked to determine if key parameters, especially, DO, ammonia, nitrite, pH and temperature, have not departed from optimal or normal conditions.
Visible examination of fresh wet preparations of skin mucus and gill tissue under a dissecting microscope or compound microscope may be sufficient to detect the presence of common parasitic infections (some fungi, protozoans and metazoans). However, identification of many pathogens, especially viruses and bacteria, requires the skill of a specialist using specialised analytical techniques. More detailed descriptions of the symptoms and diagnosis of fish diseases are found in fish health/disease texts including Ingram et al. (1991), Brown (1993), Herfort and Rawlin (1999), Noga (2000).
Decision Support Pathway For Murray Cod Health
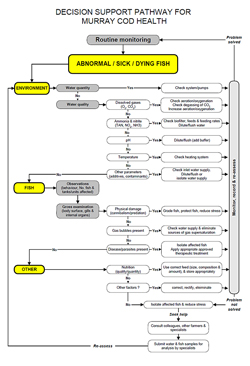
Figure 23 Decision support flowchart for identification and management of major health problems in a Murray cod aquaculture facility
Immediate action in response to a health problem will assist in reducing the severity of the incident. Where possible eliminate the probable causative agent and reduce stress. Water quality problems may be alleviated by dilution or flushing with fresh water. Infected fish should be isolated from other stock within the facility. All equipment exposed to infected/contaminated fish and water should be disinfected. Treatment of infected fish with a therapeutic drug or chemical may ultimately be necessary.
Drugs and chemicals
Aquaculturists should only use chemicals and drugs that are either registered for use by the Australian Pesticides and Veterinary Medicines Authority (APVMA) (formerly the National Registration Authority (NRA)) (http://www.apvma.gov.au), covered by a APVMA Minor use Permit or are exempt from registration. Often, use of registered chemicals, and chemicals covered by minor use permits should be used under the direction of a registered veterinarian.
Under the Chemical and Veterinary Chemicals Code Act 1994, all agricultural and veterinary chemicals (as defined by the Act) must be registered by the APVMA before they can be supplied, sold or used in Australia. Chemicals and drugs used in aquaculture may also be governed by state legislation. In recent years, efforts have been made to facilitate the registration of selected chemicals for use in Aquaculture within Australia (Percival 2001). However, today very few chemicals have been registered for use in finfish culture in Australia (see http://www.apvma.gov.au).
There are three options for registering chemicals with the APVMA. These are:
Full registration
Not usually an option in aquaculture because of the lack of data to support an application and the high costs in obtaining this data, along with the small potential market for the product. The following data is required to register new active constituents. Not as much data is needed if the product contains an approved active constituent or a product not intended for use on food producing animals. As such costs in registering a product can vary tremendously.
- Application Overview
- Chemistry & Manufacture
- Toxicology
- Metabolism & Toxicokinetics
- Residues & Overseas Trade Aspects of Residues in Food
- Occupational Health & Safety
- Environmental Studies
- Efficacy & Safety
- Other Trade Aspects
- Special Data Requirements
Permits
A permit for the use of an unregistered chemical product or an unapproved active constituent may be issued by the APVMA in certain circumstances. Permits are ideal for aquaculture because of the small quantities of the drugs / chemicals used and the lack of detailed information required for registration. Permits are divided into two main types, Off-label Permits and Trial Permits.
Off-label Permits are separated into:
Minor use:
Issued for uses not listed on the label of a product because the cost of their inclusion would exceed the economical return to its manufacturer or distributor. Examples include a product used on a specialty animal grown by a few producers on a small scale (minor animal); a product rarely used for control of a minor disease in a major animal (minor use in a major animal); or a variation made to the way in which a registered drug is administrated in special circumstances. The applicant must be able to justify to the NRA why the product and/or use should be considered under minor use permit requirements. Minor use permits are granted for a specific period and require renewal at the end of that period.
Emergency use:
An application that must be elevated rapidly to meet an actual emergency situation, such as the use of an unregistered product to control a contagious disease outbreak for which no registered product exists. Verification that the 'emergency' is genuine will be conducted by contacting the appropriate State coordinators.
Trial Permits may be issued by the NRA for small-scale trials, field trials and product evaluation trials.
Exemptions
In certain instances when a chemical is considered to be innocuous, it may not be necessary to apply for either registration or a permit. In order to apply for an exemption a letter must be submitted to the APVMA requesting the exemption, including supporting documentation to justify the request.
State 'Control of Use' Legislation
Chemicals and drugs used in aquaculture in Victoria are governed by the Agricultural and Veterinary Chemicals (Control of Use) Act 1992.
Section 6 of the act outlines offences involving use of unregistered chemical products and states that:
(1) A person must not use an unregistered chemical product unless
- the use is in accordance with a permit issued under Schedule 1; or
- the chemical product forms part of a stock of that chemical product that was in the possession of that person immediately before the chemical product ceased to be registered; and
- use of the chemical product is not prohibited under section 25A; or
- the use takes place not later than 2 years, or such other period as is determined by Order under sub-section (2), after the chemical product ceases to be registered and
- the use is by, or in accordance with the instructions of, a veterinary practitioner in the course of the practice of his or her profession and the use is not prohibited under section 25A
Legally unregistered chemicals should not be used. However, depending on individual state regulations, legal use of a chemical may also occur under the prescription of a registered veterinarian who takes responsibility for any problems or issues that may arise from the prescribed use.
Salt
Salt (sodium chloride, NaCl) is effective in controlling some external bacterial, fungal and ectoparasitic diseases in freshwater fish. Treatment concentrations range from 3-10 g/L for 30-60 minutes depending on species and age. Salt increases production of mucus and is thought to promote the healing of damaged skin and gill tissues. Salt also helps to reduce stress as it acts as a mild sedative and may benefit osmoregulation. Iodised table salt should not be used. Due to the relatively large quantities of salt that are required to treat fish diseases, its use is confined to intensive systems. Salt is generally safe and easy to use, however, it is not registered for use as a therapeutic in aquaculture by the APVMA.
Further information:
Selosse and Rowland 1990; Harrell 1992.
Formalin
Formalin (37% by weight formaldehyde) is used extensively throughout the aquaculture industry as a treatment of certain diseases in aquaculture, especially external bacterial, fungal, protozoan and metazoan diseases. Formalin is registered under a minor use permit for native finfish. High concentrations are used to treat fungal infections on eggs. Since formalin is a known carcinogen and formaldehyde is a noxious gas, this chemical should be handled with caution and used according to the supplier's instructions. Since formalin chemically removes oxygen from water (each 5 mg/L formalin removes 1 mg/L dissolved oxygen), systems being treated should be well aerated. Formalin toxicity increases with increasing temperature. Sometimes a white/cloudy precipitate, known as paraformaldehyde, develops in formalin that has been stored for long periods or been exposed to cold temperatures. Paraformaldehyde is highly toxic to fish and should not be used in treatments. To remove paraformaldehyde, decant the clear formalin into another container and discard the remaining precipitate.
Further information:
Francis-Floyd 1992; Howe et al. 1995.
Chemicals and drugs management
Chemicals, therapeutics and medicines should be stored in dry facilities to avoid degradation of the substances or their packaging. Stores should be secured to stop access by foraging animals and unauthorised personnel. Farmers are advised to follow either label directions provided by the manufacturers or suppliers, or guidelines provided by a suitably qualified veterinarian or specialist (or the APVMA for minor use permits). Disposal of chemicals and management of chemical spills should be undertaken in accordance with guidelines and recommendations provided by the manufacturers (i.e. material safety data sheets).
Acknowledgements
The authors wish to acknowledge the following persons for their assistance in the preparation of this document. Financial support was provided by The Victorian Fisheries Authority and the Fisheries Research and Development Corporation (FRDC) (via Project No. 1999/328). The following persons are acknowledged for providing images. Faulding Images for microscopes (Figure 1). Dr Wendy Townsend (Animal Research Institute, Yeerongpilly, Qld) for Clinostomum complanatum (photographed by Peter Green) (Figure 12). Phil Read (NSW DPI - Fisheries, Grafton, NSW) for silver perch infected with Epizootic Ulcerative Syndrome on (Figure 4). Bob Collins (Deakin University, Warrnambool, Vic) for Histiostoma papillata (Figure 15). Geoff Gooley (Department of Primary Industries, Queenscliff, Vic.) for Swollen yolk-sac syndrome (Figure 17a). Janine Muller (Department of Primary Industries, Attwood, Vic.) for chronic erosive dermatopathy (Figure 22). The authors wish to thank the following people for providing comments on various drafts of the document. Crispian Ashby (FRDC, Canberra), Anthony Forster (The Victorian Fisheries Authority, Melbourne) Simon and Phillippa Noble (Brimmin Lodge, Rutherglen), Steve and Catherine Kanara (L.V. Recirc, Traralgon), Helen O'Brien (Department of Primary industries, Snobs Creek), Geoff Gooley (Department of Primary industries, Queenscliff) and participants from the Victorian fish farming industry that attended a fish health workshop at Snobs Creek (31 May 2002).
References
General fish health references
Austin, B. and Austin, D.A. (1993). Bacterial Fish Pathogens. Disease in Farmed and Wild Fish. Ellis Horwood Ltd., New York. 384 pp.
Brune, D.E. and Tomasso, J.R. (Eds.) (1991). Aquaculture and Water Quality. The World Aquaculture Society, Baton Rouge. 606 pp.
Bryden, D.I. (Ed.) (1988). Fish Diseases. Refresher Course for Veterinarians. Proceedings 106 (23-27 May, 1988, Sydney). Post Graduate Committee in Veterinary Science University of Sydney, Sydney. 635 pp.
De Silva, S.S. and Anderson, T.A. (1995). Fish Nutrition in Aquaculture. Chapman and Hall, Melbourne. 319 pp.
Goddard, S. (1996). Feed Management in Intensive Aquaculture. Chapman and Hall, New York. 194 pp.
Herfort, A. and Rawlin, G. (1999). The Australian Aquatic Animal Disease Identification Field Guide. Agriculture, Fisheries and Forestry, Canberra. 91 pp.
Humphrey, J.D. and Langdon, J.S. (Eds.) (1985).Proceedings of the Workshop on Diseases of Australian Fish and Shellfish (27-30 May 1985, Benalla, Victoria). Australian Fish Health Reference Laboratory, Benalla. 311 pp.
Hoffman, G.L. and Meyer, F.P. (1974). Parasites of Freshwater Fishes. A Review of Their Control and Treatment. T.F.H. Publications, Neptune City. 224 pp.
Iwama, G.K., Pickering, A.D., Sumpter, J.P. and Schreck, C.B. (Eds.) (1997). Fish Stress and Health in Aquaculture. Society for Experimental Biology Seminar Series 62. Cambridge University Press, Cambridge. 278 pp.
MacMillan, J.R. (1991). Biological factors impinging upon control of external protozoan fish parasites. Annual Review of Fish Diseases: 119-131.
Munday, B. (Ed.) (1990). Fin Fish Diseases. Refresher Course for Veterinarians. Proceedings 128 (26-28 January, 1990, Launceston, Tasmania). Post Graduate Committee in Veterinary Science University of Sydney, Sydney. 290 pp.
Munday, B. (Ed.) (1992). Fin Fish Workshop. Refresher Course for Veterinarians. Proceedings 182 (21-26 January, 1992, Launceston, Tasmania). Post Graduate Committee in Veterinary Science University of Sydney, Sydney. 357 pp.
Noga, E.J. (2000). Fish Disease Diagnosis and Treatment. Iowa State University, Ames, Iowa. 367 pp.
Paperna, I. (1991). Diseases caused by parasites in the aquaculture of warm water fish. Annual Review of Fish Diseases: 155-194.
Sarig, S. (1971). Diseases of Fishes. Book 3: The Prevention and Treatment of Diseases of Warmwater Fishes under Subtropical Conditions, with Special Emphasis on Intensive Fish Farming. TFH Publications, Inc. Ltd., London.
Schlotfield, H.-J. and Alderman, D.J. (1995). What Should I do? A Practical Guide for the Fresh Water Fish Farmer. Supplement to Bulletin of The European Association of Fish Pathologists 15(4): 60 pp.
References cited in text
Alderman, D.J., and Hastings, T.S. (1998). Antibiotic use in aquaculture: development of antibiotic resistance - potential for consumer health risks. International Journal of Food Science and Technology 33(2): 139-155.
Anon. (1999). Aquaplan. Australia's National Strategic Plan for Aquatic Animal Health 1998-2003.
National Office of Animal and Plant Health, Agriculture, Fisheries and Forestry - Australia, Canberra. 33 pp.
Anon. (2001). Possible eye damage associated with fluke infections in Murray cod (Maccullochella peeli). Queensland Government Department of Primary Industries Fish Disease Report 2(July): 4-5.
ANZECC. (1999). Water Quality Guidelines for the Protection of Aquatic Food Species.
ANZECC. (2000). Water quality guidelines for the protection of cultured fish, molluscs and crustaceans. In: Australian and New Zealand Guidelines for Fresh and Marine Water Quality. Paper No. 4 - Volume 3 (Chapter 9). Australian and New Zealand Environment and Conservation Council, Agriculture and Resource Management Council of Australia and New Zealand. Pp 11-63.
Ashburner, L.D. (1978). Management and diseases of hatchery fish. In: University of Sydney Post-Graduate Committee in Veterinary Sciences. Refresher Courses for Veterinarians Proceedings. Vol. 36. Pp 387-449.
Ashburner, L.D., and Ehl, A.S. (1973). Chilodonella cyprini (Moroff), a parasite of freshwater fish and its treatment. Australian Society for Limnology Bulletin 5: 3-4.
Baily, J.E. (2003). A Histopathology based Investigation of Chronic Erosive Dermatopathy in Murray cod, Maccullochella peelii peelii. Masters Thesis. University of Stirling, Stirling. 146 pp.
Baily, J.E., Bretherton, M.J., Gavine, F.M., Ferguson, H.W., and Turnbull, J.F. (2005). The pathology of chronic erosive dermatopathy in Murray cod, Maccullochella peelii peelii (Mitchell). Journal of Fish Diseases 28(1): 3-12.
Barlow, C.G. (1995). Bird predation of silver perch in ponds. In: S.J. Rowland and C. Bryant (Eds), Silver Perch Culture. Proceedings of Silver Perch Workshops, Grafton and Narrandera, April 1994. Austasia Aquaculture, Sandy Bay, Tasmania. Pp 89-96.
Becker, C.D., and Kreier, J.P. (1977). Flagellate parasites of fish. In: C.D. Becker and J.P. Kreier (Eds), Parasitic Protozoa. Volume 1. Taxonomy, kinetoplastids, and flagellates of fish. 1977, 357-416.Academic Press Inc., London. Pp 357-416.
Bernoth, E.M., Murray, G., Rickard, M.D., and Hurry, G. (1999). Approaches to managing aquatic animal health in Australia. Revue Scientifique et Technique de L Office International Des Epizooties 18(1): 228-238.
Beumer, J.P., Ashburner, L.D., Burbury, M.E., Jetté, E., and Latham, D.J. (1982). A Checklist of the Parasites of Fishes from Australia and its Adjacent Antarctic Territories. Commonwealth Agricultural Bureaux, Slough, England. 99 pp.
Bisogni, J.J., and Timmons, M.B. (1994). Control of pH in closed cycle aquaculture systems. In: M.B. Timmons and T.M. Losordo (Eds), Aquaculture Water Reuse Systems: Engineering Design and Management. Elsevier, New York. Pp 235-245.
Boreham, S., Ingram, B.A., Jones, P.L., and Collins, R.O. (2004). Water quality and intensive Murray cod aquaculture. In: B.A. Ingram and S.S. De Silva (Eds), Development of Intensive Commercial Aquaculture Production Technology for Murray cod. Final Report to the Fisheries Research and Development Corporation (Project No. 1999/328). Primary Industries Research Victoria, DPI, Alexandra, Victoria, Australia. Pp 113-128.
Boyd, C.E. (1990). Water Quality in Ponds for Aquaculture. Alabama Agricultural Experiment Station, Auburn University, Auburn University, Alabama. 482 pp.
Brown, J.H. (1989). Antibiotics: their use and abuse in aquaculture. World Aquaculture 20(2): 34 43.
Brown, L. (Ed) (1993). Aquaculture for Veterinarians. Fish Husbandry and Medicine. Pergamon Press Ltd., Oxford. 447 pp.
Bryden, D.I. (Ed) (1988). Fish Diseases. Refresher Course for Veterinarians. Post Graduate Committee in Veterinary Science University of Sydney, Sydney. 635 pp.
Callinan, R.B., and Rowland, S.J. (1995). Diseases of silver perch. In: S.J. Rowland and C. Bryant (Eds), Silver Perch Culture. Proceedings of Silver Perch Workshops, Grafton and Narrandera, April 1994. Austasia Aquaculture, Sandy Bay, Tasmania. Pp 67-75.
Callinan, R.B., Rowland, S.J., Jiang, L., and Mifsud, C. (1999). Outbreaks of epizootic ulcerative syndrome (EUS) in farmed silver perch Bidyanus bidyanus in New South Wales, Australia. In: The Annual International Conference and Exposition of the World Aquaculture Society. Book of Abstracts. (26 April - 2 May 1999), Sydney. P. 127.
Carson, J. (1990). Bacterial infections of fish. In: B.L. Munday (Ed) Fin Fish Diseases. Refresher Course for Veterinarians. Proceedings 128 (26-28 January 1990, Launceston, Tasmania). Post Graduate Committee in Veterinary Science University of Sydney, Sydney. Pp 56-72.
Carson, J. (1992). New bacterial diseases of fish. In: B. Munday (Ed) Fin Fish Workshop. Refresher Course for Veterinarians. Proceedings 182 (21-26 January 1992, Launceston, Tasmania). Post Graduate Committee in Veterinary Science University of Sydney, Sydney. Pp 315-333.
Coulibaly, N.D., Salembere, S., and Bessin, R. (1995). (Larval clinostomosis in cichlid fish in Lake Kompienga in Burkina Faso: a threat to fishing and public health). Cahiers-d'Etudes-et-deRecherches-Francophones-Sante 5(3): 189-193.
Crane, M.S., Hardy-Smith, P., Williams, L.M., Hyatt, A.D., Eaton, L.M., Gould, A., Handlinger, J., Kattenbelt, J., and Gudkovs, N. (2000). First isolation of an aquatic birnavirus from farmed and wild fish species in Australia. Diseases of Aquatic Organisms 43(1): 1-14.
Cribb, T.H. (1988). Two new digenetic trematodes from Australian fishes with notes on previously described species. Journal of Natural History 22(1): 27-43.
Dixon, B.A. (1994). Antibiotic resistance of bacterial fish pathogens. Journal of the World Aquaculture Society 25(1): 60-63.
El-Matbouli, M., Fischer-Scherl, T., and Hoffman, R.W. (1992). Present knowledge on the life cycle, taxonomy, pathology, and therapy of some Myxosporea spp. important for freshwater fish. Annual Review of Fish Diseases: 367-402.
Emerson, K., Russo, R.C., Lund, R.E., and Thurston, R.V. (1975). Aqueous ammonia equilibrium calculations: effect of pH and temperature. Journal of the Fisheries Research Board of Canada 32: 2379-2383.
Ferguson, H.W. (1988). Nutritional diseases. In: D.I. Bryden (Ed) Fish Diseases. Refresher Course for Veterinarians Proceedings 106 (23-27 May, 1988, Sydney). Post Graduate Committee in Veterinary Science University of Sydney, Sydney. Pp 87-93.
Flick, G.J. (1998). Common chemicals for cleaning and disinfecting aquaculture facilities. In: G.S. Libey and M.B. Timmons (Eds), Proceedings of the Second International Conference on Recirculating Aquaculture. Virginia Polytechnic Institute and State University, Roanoke, Virginia. Pp 7-19.
Francis-Floyd, R. (1992). Use of formalin to control fish parasites. Institute of Food and Agricultural Sciences, University of Florida.
Glazebrook, J.S., Heasman, M.P., and de Beer, S.W. (1990). Picorna-like viral particles associated with mass mortalities in larval barramundi, Lates calcarifer Bloch. Journal of Fish Diseases 13: 245-249.
Goddard, S. (1996). Feed Management in Intensive Aquaculture. Chapman and Hall, New York. 194 pp.
Grace, G.R., and Piedrahita, R.H. (1994). Carbon dioxide control. In: M.B. Timmons and T.M. Losordo (Eds), Aquaculture Water Reuse Systems: Engineering Design and Management. Elsevier, New York. Pp 209-234.
Gunasekera, R.M., Gooley, G.J., and De Silva, S.S. (1998). Characterisation of 'swollen yolk-sac syndrome' in the Australian freshwater fish Murray cod, Maccullochella peelii peelii, and associated nutritional implications for large scale aquaculture. Aquaculture 169(1-2): 69-85.
Halliday, R.B., and Collins, R.O. (2002). Histiostoma papillata sp. n. (Acari: Histiostomatidae), a mite attacking fish in Australia. Australian Journal of Entomology 41: 155 - 158.
Hargis, W.J. (1991). Disorders of the eye in finfish. Annual Review of Fish Diseases: 95-117.
Harrell, R.M. (1992). Stress mitigation by use of salt and anaesthetic for wild striped bass captured for brood stock. Progressive Fish-Culturist 54: 228-233.
Hecht, T., and Pienaar, A.G. (1993). A review of cannibalism and its implications in fish larviculture. Journal of the World Aquaculture Society 24(2): 246-261.
Herfort, A., and Rawlin, G. (1999). The Australian Aquatic Animal Disease Identification Field Guide. Agriculture, Fisheries and Forestry, Canberra. 91 pp.
Hoffman, G.L. (1978). Ciliates of freshwater fishes. In: J.P. Kreier (Ed) Parasitic Protozoa. Vol. 2. Academic press, New York, San Francisco, London. Pp 573-632.
Howe, G.E., Marking, L.L., Bills, T.D., and Schreier, T.M. (1995). Efficacy and toxicity of formalin solutions containing paraformaldehyde for fish and egg treatments. Progressive Fish-Culturist 57: 147-152.
Humphrey, J., Muller, J., and Lancaster, M. (2000). Chronic erosive dermatopathy in Murray cod. In: B.A. Ingram (Ed) Murray Cod Aquaculture A Potential Industry for the New Millennium. Proceedings of a Workshop (18th January 2000, Eildon, Vic.). Marine and Freshwater Resources Institute, Alexandra. Pp 35.
Humphrey, J.D., and Langdon, J.S. (Eds). (1985). Proceedings of the Workshop on Diseases of Australian Fish and Shellfish (27-30 May 1985, Benalla, Victoria). Australian Fish Health Reference Laboratory, Benalla. 311 pp.
Ingram, B. (1997). The diversity and complexity of aquatic plants and animals in aquaculture ponds. Austasia Aquaculture 11(1): 54-58.
Ingram, B., and Larkin, B. (2000). Murray cod aquaculture - current information and current status. In: B.A. Ingram (Ed) Murray Cod Aquaculture A Potential Industry for the New Millennium. Proceedings of a Workshop (18th January 2000, Eildon, Vic.). Marine and Freshwater Resources Institute, Alexandra. Pp 4-16.
Ingram, B.A. (Ed) (2000). Murray Cod Aquaculture A Potential Industry for the New Millennium. Proceedings of a Workshop (18th January 2000, Eildon, Vic.). Marine and Freshwater Resources Institute, Alexandra. 43 pp.
Ingram, B.A. (2001). Rearing Juvenile Australian Native Percichthyid Fish in Fertilised Earthen Ponds. PhD Thesis. Deakin University, Warrnambool. 224 pp.
Ingram, B.A. (2004). Murray cod fingerling production and growout. In: B.A. Ingram and S.S. De Silva (Eds), Development of Intensive Commercial Aquaculture Production Technology for Murray cod. Final Report to the Fisheries Research and Development Corporation (Project No. 1999/328). Primary Industries Research Victoria, DPI, Alexandra, Victoria, Australia. Pp 25-65.
Ingram, B.A., Barlow, C.G., Burchmore, J.J., Gooley, G.J., Rowland, S.J., and Sanger, A.C. (1990). Threatened native freshwater fishes in Australia - some case histories. Journal of Fish Biology 37 (Supplement A): 175-182.
Ingram, B.A., and De Silva, S.S. (Eds). (2004). Development of Intensive Commercial Aquaculture Production Technology for Murray cod. Final Report to the Fisheries Research and Development Corporation (Project No. 1999/328). Primary Industries Research Victoria, DPI, Alexandra, Victoria, Australia. 202 pp.
Ingram, B.A., Gavine, F., and Lawson, P. (2004). Diseases and health management in intensive Murray cod aquaculture. In: B.A. Ingram and S.S. De Silva (Eds), Development of Intensive Commercial Aquaculture Production Technology for Murray cod. Final Report to the Fisheries Research and Development Corporation (Project No. 1999/328). Primary Industries Research Victoria, DPI, Alexandra, Victoria, Australia. Pp 129-146.
Ingram, B.A., Hawking, J.H., and Shiel, R.J. (1997). Aquatic life in freshwater ponds. A guide to the identification and ecology of life in aquaculture ponds and farm dams in south eastern Australia. Cooperative Research Centre for Freshwater Ecology, Albury. 105 pp.
Ingram, B.A., Missen, R., and Dobson, J.L. (2001). Best Practice Guidelines for Weaning Pond-reared Murray Cod Fingerlings onto an Artificial Diet Report No. 36. Marine and Freshwater Resources Institute. Report No. 36, Alexandra. 7 pp.
Ingram, B.A., and Philbey, A.W. (1999). Occurrence of the parasite Ergasilus intermedius (Copepoda: Ergasilidae) on the gills of Macquarie perch, Macquaria australasica (Percichthyidae). Proceedings of the Linnean Society of New South Wales. NSW. 121: 39-44.
Isobe, A., Kinoshita, S., Hojo, N., Fukushima, T., Shiwaku, K., and Yamane, Y. (1994). The 12th human case of Clinostomum sp. infection in Japan. Japanese Journal of Parasitology 43(3): 193-198.
Iwama, G.K., Pickering, A.D., Sumpter, J.P., and Schreck, C.B. (Eds). (1997). Fish Stress and Health in Aquaculture. Cambridge University Press, Cambridge. 278 pp.
Johnston, T.H., and Angel, L.M. (1941). Life cycle of the trematode (Diplostomum Murrayense) J.& C. Transactions of the Royal Society of South Australia 65(1): 140-144.
Johnston, T.H., and Mawson, P.M. (1940). Some nematodes parasitic in Australian freshwater fish. Transactions of the Royal Society of South Australia 64(2): 340-351.
Johnston, T.H., and Mawson, P.M. (1947). Some avian and fish nematodes, chiefly from Tailem Bend, South Australia. Records of the S.A. Museum 28(4): 547-53.
Johnston, T.H., and Mawson, P.M. (1951). Additional nematodes from Australian fish. Transactions of the Royal Society of South Australia 74(4): 18-24.
Kabata, Z. (1992). Copepoda parasitic on Australian fishes, XV. Family Ergasilidae (Poecilostomatoida). Journal of Natural History 26: 47-66.
King, R.K., and Flick, G. (2000). The potential for the presence of bacterial pathogens in biofilms of recirculating aquaculture systems. In: G.S. Libey and M.B. Timmons (Eds), Proceedings of the Third International Conference on Recirculating Aquaculture. Virginia Polytechnic Institute and State University, Roanoke, Virginia. Pp 335-347.
Lancaster, M.J., Williamson, M.M., and Schroen, C.J. (2003). Iridovirus-associated mortality in farmed Murray cod (Maccullochella peelii peelii). Australian Veterinary Journal 81(10): 633-634.
Langdon, J.S. (1989). Experimental transmission and pathogenicity of epizootic haematopoietic necrosis virus (EHNV) in redfin perch, Perca fluviatilis L., and 11 other teleosts. Journal of Fish Diseases 12(4): 295-310.
Langdon, J.S., Humphrey, J.D., and Williams,L.M. (1986). First virus isolation from Australian fish: an iridovirus-like pathogen from redfin perch, Perca fluviatilis L. Journal of Fish Diseases 9: 263-268.
Langdon, J.S., Humphrey, J.D., and Williams, L.M. (1988). Outbreaks of an EHNV-like iridovirus in cultured rainbow trout, Salmo gairdneri Richardson, in Australia. Journal of Fish Diseases 11: 93-96.
Larkin, B. (2000). Recirculating aquaculture systems in Australia: A synthesis of systems, species, and the networking approach. In: G.S. Libey, M.B. Timmons, G.J. Flick, and T.T. Rakestraw (Eds), Proceedings of the Third International Conference on Recirculating Aquaculture. Virginia Polytechnic Institute and State University, Roanoke, Virginia. Pp 251-266.
Lilley, J.H., Callinan, R.B., Chinabut, S., Kanchanakhan, S., MacRae, I.H., and Phillips, M.J. (1998). Epizootic Ulcerative Syndrome (EUS) Technical Handbook. The Aquatic Animal Health Research Institute, Bangkok. 88 pp.
McKinnon, L., and Shepheard, N. (1995). Factors contributing to a fish kill in Broken Creek. Victorian Naturalist 112(2): 93-99.
Missen, R., and Dobson, J. (2000). Parasitic diseases of juvenile Murray cod in hatchery facilities. In: B.A. Ingram (Ed) Murray Cod Aquaculture A Potential Industry for the New Millennium. Proceedings of a Workshop (18th January 2000, Eildon, Vic.). Marine and Freshwater Resources Institute, Alexandra. Pp 37-41.
Molnar, K., and Rohde, K. (1988). New coccidians from freshwater fishes of Australia. Journal of Fish Diseases 11: 161-169.
Munday, B. (Ed) (1990). Fin Fish Diseases. Refresher Course for Veterinarians. Post Graduate Committee in Veterinary Science University of Sydney, Sydney. 290 pp.
Munday, B. (Ed) (1992). Fin Fish Workshop. Refresher Course for Veterinarians. Post Graduate Committee in Veterinary Science University of Sydney, Sydney. 357 pp.
Munday, B.L. (1989). Bacterial diseases of fish. In: Fish diseases. University of Sydney, Sydney. Pp 101-110.
Neish, G.A., and Hughes, G.C. (1980). Diseases of Fishes. Book 6: Fungal Diseases of Fishes. TFH Publications, Hong Kong. 155 pp.
Noga, E.J. (2000). Fish Disease Diagnosis and Treatment. Iowa State University, Ames, Iowa. 367 pp.
OIE. (2001). International Aquatic Animal Health Code. Fish, Molluscs and Crustaceans. 4th Edition. Office International des Epizooties, Paris. 153 pp.
Paperna, I. (1991). Diseases caused by parasites in the aquaculture of warm water fish. Annual Review of Fish Diseases 1991: 155-194.
Percival, S. (2001). Registration of Aquaculture Chemicals. Project 96/314. Aquaculture Development and Veterinary Services Pty Ltd (ADVS) and Fisheries Research and Development Corporation, Blackmans Bay, Tasmania. 121 pp.
Philbey, A.W., and Ingram, B.A. (1991). Coccidiosis due to Goussia lomi (Protista: Apicomplexa) in aquarium-reared Murray cod, Maccullochella peeli (Mitchell), (Percichthyidae). Journal of Fish Diseases 14: 237-242.
Piper, R.G., McElwain, I.B., Orme, L.E., McCraren, J.P., Fowler, L.G., and Leonard, J.R. (1998). Fish Hatchery Management. US Department of the Interior, Fish and Wildlife Service, Washington, D.C. 517 pp.
Robb, D. (2000). Humane slaughter. In: Proceedings of the British Trout Farming Conference (5-7 September 2000, Sparsholt College, Hampshire, England). British Trout Farming Association. Pp 57-64.
Roberts, R.J. (Ed) (1982). Microbial Diseases of Fish. Academic Press, London. 305 pp.
Rowland, S.J. (1995). Water quality in the intensive culture of silver perch. In: S.J. Rowland and C. Bryant (Eds), Silver Perch Culture. Proceedings of Silver Perch Workshops, Grafton and Narrandera, April 1994. Austasia Aquaculture, Sandy Bay, Tasmania. Pp 51-65.
Rowland, S.J., and Ingram, B.A. (1991). Diseases of Australian native freshwater fishes with particular emphasis on the ectoparasitic and fungal diseases of Murray cod (Maccullochella peeli), golden perch (Macquaria ambigua) and silver perch (Bidyanus bidyanus). NSW Fisheries, Sydney. 33 pp.
Sarig, S. (1971). Diseases of Fishes. Book 3: The Prevention and Treatment of Diseases of Warmwater Fishes under Subtropical Conditions, with Special Emphasis on Intensive Fish Farming. TFH Publications, Inc. Ltd., London.
Selosse, P.M., and Rowland, S.J. (1990). Use of common salt to treat ichthyophthiriasis in Australian warmwater fishes. The Progressive Fish-Culturist 52(2): 124-127.
Shepherd, J., and Bromage, N. (Eds). (1988). Intensive Fish Farming. BSP Professional Books, Oxford. 404 pp.
Skrudland, A. (1993). Nutrition in aquaculture. In: L. Brown (Ed) Aquaculture for Veterinarians. Fish Husbandry and Medicine. Pergamon Press, Oxford. Pp 153-159.
Smith, C.E., and Reay, P. (1991). Cannibalism in teleost fish. Reviews in Fish Biology & Fisheries 1: 41-64.
Sorensen, E.M. (1991). Metal Poisoning in Fish. CRC Press, Boca Raton, Florida. 383 pp.
Thoney, D.A., and Hargis, W.J.J. (1991). Monogenea (Platyhelminthes) as hazards for fish in confinement. Annual Review of Fish Diseases 1991: 133-153.
Trott, P. (2000). Investigation of the characteristic causes for chronic erosive dermatopathy in Murray cod. In: B.A. Ingram (Ed) Murray Cod Aquaculture A Potential Industry for the New Millennium. Proceedings of a Workshop (18th January 2000, Eildon, Vic.). Marine and Freshwater Resources Institute, Alexandra. Pp 36.
Trott, P.J. (1999). An investigation of the characteristic causes for chronic erosive dermatopathy found in Murray cod, Maccullochella peelii peelii (Percichthyidae), within intensive aquaculture facilities throughout Victoria and New South Wales. Honours Thesis. Deakin University, Warrnambool. 75 pp.
Whittington, R.J., Gudkovs, N., Carrigan, M.J., Ashburner, L.D., and Thurstan, S.J. (1987). Clinical, microbiological and epidemiological findings in recent outbreaks of goldfish ulcer disease due to atypical Aeromonas salmonicida in south-eastern Australia. Journal of Fish Diseases 10: 353-362.
Appendix 1 - Monitoring guidelines
Parameter | Frequency of sampling | Comments |
---|---|---|
General (all data sheets) | ||
Pond/tank number | Every sample period | |
Date and time | Every sample period | |
Primary water quality parameters | ||
Temperature | Daily | May fluctuate diurnally |
Dissolved oxygen | 1-2 daily (RAS) | Marked diurnal fluctuations can occur in ponds |
1-3 weekly (ponds) | ||
pH | 1-2 daily (RAS) | Marked diurnal fluctuations can occur in ponds |
1-3 weekly (ponds) | ||
Ammonia | Daily (RAS) | Amount of ammonia in toxic form influenced by temperature and pH |
Weekly (ponds) | ||
Nitrite | Daily (RAS) | |
Weekly (ponds) | ||
Nitrate | Daily (RAS) | |
Weekly (ponds) | ||
Other water quality parameters | ||
Alkalinity | Weekly (ponds) | Where water source is low in alkalinity |
Phosphorus or phosphate | Weekly (ponds) | |
Secchi disk depth | Daily to weekly (ponds) | Indicator of plankton density |
Turbidity | As required | |
Suspended solids | As required | |
Salinity | As required | |
Fish | ||
Sample collection | 1-2 weekly | |
Behaviour | Each sampling | Feeding activity swimming behaviour etc. |
Appearance | Each sampling | Skin, eyes, fins, gills, colouration, markings, fin condition, shape of body etc. |
No. fish affected | Each sampling | No. of moribund fish, No. of dead fish, etc. |
No. fish sampled | Each sampling | |
Length of fish | Each sampling | |
Weight of fish | Each sampling | |
Parasite species observed | Each sampling | If cannot be identified, photograph and/or draw and describe size, appearance and behaviour. |
Location of parasites on/in fish | Each sampling | body surface, gills, fins, internal organs |
No. of parasites present | Each sampling | No. observed per field of view |
Action taken | Each sampling | What action was taken following inspection of fish (ie, treatments applied et.) |
Additional useful information | ||
Tank/pond volume, date filled, date stocked | ||
Source/Species/strain of fish stocked | ||
Length, weight and age at stocking,and Number fish stocked | ||
Feeding information (Feed type and feed rate etc) | ||
Chemicals added (type amount and reason for addition) |
Appendix 2 - Notifiable fish diseases
Diseases notifiable to the Office International des Epizooties (OIE) are considered to be of socioeconomic and/or public health importance, and that are significant in the international trade of aquatic animals and aquatic animal products (OIE 2001) (see table below).
Supplementary to the notifiable diseases, the OIE lists "other significant diseases". These diseases are of current or potential international significance in aquaculture but are either:
- less important than notifiable disease, or
- have a limited geographic distribution, or
- a geographic distribution so wide the notification is not meaningful, or
- the disease is not currently sufficient investigated and understood.
The diseases listed by the OIE represent Australia's international notification and or reporting obligations.
Within Australia, lists of notifiable or reportable fish diseases are defined by each state (see table below). These lists include all relevant OIE listed notifiable diseases. In addition, these lists may include diseases that are listed as "other significant diseases" by the OIE due to the state/regional/Local distribution and or potential impact within the state. In Victoria notifiable diseases for aquatic animals are listed under both the Fisheries Regulations 1998 (Schedule 16) and the Livestock Disease Control Regulations 1995 (Schedule 2 Table 3).
Pursuant to Section 52 of the Fisheries Act 1995, in addition to any condition imposed by the act, a fishery licence or a permit is subject to:
(a) any conditions that the Secretary thinks is appropriate and that are expressed or referred to in the licence or permit and
(b) any conditions that are set out in the regulations. The following regulation specifies the requirements in the event of a notifiable disease outbreak in Victoria:
Regulation 335 (2) states that the licence holder -
(d)(i) must within 24 hours of the outbreak of any notifiable disease in an aquaculture crop notify the Secretary by telephone; and,
(ii) must within 5 days of the outbreak of the notifiable disease in the crop, notify the Secretary in writing.
(e) must not sell remove, discharge, dispose of or transfer any fish, seawater, or equipment from an area that is affected by notifiable disease or toxic algae to any other area.
In summary, this means that the Secretary must be notified of an outbreak of any disease as listed in Schedule 16 by phone within 24 hours and in writing within five days.
In addition, a person must not transport stock from an area affected by a notifiable disease. This has two implications:
- a farmer should inquire as to the disease status of all purchased stock and the disease status in the area. It would be advisable to have stock certified as free from diseases of concern.
- a farmer must not move stock or equipment from one affected area to another. This potentially includes the movement of infected stock around the premises eg from tanks to ponds.
Notifiable and reportable diseases
Disease | Office International des Epizootics (OIE) | Department of Agriculture, Fisheries & Forestry - Australia (AFFA) | VICTORIA |
---|---|---|---|
Epizootic haematopoietic necrosis (EHN)1* | Notifiable | Reportable | Notifiable |
Infectious haematopoietic necrosis | Notifiable | Reportable | Notifiable |
Oncorhynchus masou virus disease | Notifiable | Reportable | Notifiable |
Spring viraemia of carp | Notifiable | Reportable | Notifiable |
Viral haemorrhagic septicaemia | Notifiable | Reportable | Notifiable |
Channel catfish virus diseases | Other significant | Reportable | Notifiable |
Viral encephalopathy and retinopathy1 | Other significant | Reportable | Notifiable |
Infectious pancreatic necrosis | Other significant | Reportable | Notifiable |
Infectious salmon anaemia | Other significant | Reportable | Notifiable |
Epizootic ulcerative syndrome (EUS)1 | Other significant | Reportable | Notifiable |
Bacterial Kidney Disease (Renibacterium salmoninarum) | Other significant | Reportable | Notifiable |
Enteric septicaemia of catfish (Edwardsiella ictaluri) | Other significant | Reportable | Notifiable |
Piscirickettsiosis (Piscirickettsia salmonis) | Other significant | Reportable | Notifiable |
Gyrodactylosis (Gyrodactylus salaris) | Other significant | Reportable | Notifiable |
Red sea bream iridoviral disease | Other significant | ||
White sturgeon iridoviral disease | Other significant | ||
Aeromonas salmonicida (atypical strains) (GUD)1* | Reportable | Notifiable | |
Aeromonas salmonida var. salmonicida (furunculosis) | Reportable | Notifiable | |
Whirling disease | Reportable | Notifiable | |
Enteric redmouth diesease (Yersinia ruckeri) | Reportable | Notifiable | |
Barramundi picorna-like virus1 | Notifiable |
1. Recorded from Australia but restricted to some areas.
* Murray cod experimentally infected in laboratory trials only
Appendix 3 - Submission of samples for disease diagnosis
Contact a fish health specialist and/or fish veterinary service prior to preparing samples for dispatch. Some tests undertaken by the specialists may need prior notice.
At this stage it is useful to have made some preliminary observations on the disease situation which can be discussed with the specialist including:
- Species of fish and number of fish affected
- Symptoms observed (fish behaviour, signs of disease etc)
- Prevailing environmental conditions (water quality etc)
- Previous treatments undertaken.
If samples are to be sent for examination. Request information on cost of services, what "tests" will be undertaken, what specimens should be sent and how the samples should be prepared for dispatch.
All specimens should be clearly labelled with the following information:
-Name, address and contact details of person requesting the examination
-Description of contents of the consignment including species, numbers, and method of preservation.
- A description of observations made
Methods of submission. Accurate diagnosis of a disease is largely dependant on the quality of the specimens submitted for examination, and the accompanying documentation describing the event. The type of examination and tests that can be undertaken will also depend on the way specimens are submitted. Live, freshly killed on ice specimens should reach the laboratory within 24 hours of dispatch. Preferably a number of specimens should be submitted including moribund fish and fish exhibiting different stages of the disease. Parasites are more easily isolated and identified when living.
Live specimens are preferred as they allow for more flexibility in diagnosis options by the specialist. Place the fish in a strong plastic bag (or two). Ensure that sufficient packaging procedures are used that the fish will survive the transport period (24 hours). Consideration should be given to time in transit, number fish, and volume of water and air/oxygen.
Diagnosis procedure | Method of submission | |||
---|---|---|---|---|
Live | Freshly killed on ice | Frozen | Preserved in a fixative | |
Gross examination | YES | YES | YES | YES |
Toxicology | YES | YES | YES | |
Parasitology | YES | YES | Limited | Limited |
Bacteriology | YES | YES | ||
Virology | YES | YES | ||
Pathology | YES | YES |
No more than half the volume of the container should be water to ensure there is a sufficient amount of air/oxygen above the water to maintain dissolved oxygen levels. Bags should be sealed to prevent air and water leakage and containers should be insulated to reduce temperature changes.
Often dead specimens are less useful because the rapid post-mortem invasion of bacteria and degeneration of tissues reduce the usefulness of sample for virology, bacteriology and pathology. Some external parasites may quickly leave the fish soon after death. Dead fish should be placed in clean sealed plastic bags and placed in an esky containing crushed ice. Ensure that water leakage cannot occur.
Send frozen samples as a last resort. The ability to detect viruses, bacteria and parasites in these samples is greatly reduced or impossible in many cases. Further, subsequent thawing of samples causes cellular disruption which makes histopathology ineffective. Samples should be sealed in clean plastic bags and snap frozen. These samples can then be sent in an insulated container with crushed ice. Ensure that samples will reach their destination before thawing occurs, and that leakage cannot occur.
Samples may also be submitted in a preservative such as formalin (see below). To ensure rapid preservation of internal organs, slit open the body cavity of larger fish before placing into the preservative. Care should be taken to avoid damage to internal organs. Ensure that excess preserving solution is used. The biomass of fish being preserved should take up less than 25% of the volume of the preserving agent. It is prudent to preserve some fish even if live samples are being submitted as this is insurance against loss due to delays in transport resulting in death and/or deterioration of live fish or samples sent on ice. Preservative containers must be leakproof and ideally made of non-brittle plastic to avoid breakage during transit. As a precaution seal the containers in bottles in plastic bags and ensure adequate package is used to prevent damage.
Fixative for preserving samples:
10% neutral buffered formalin in saline: | |
100ml | concentrated formalin (40% w/v formaldehyde) |
900 ml | tap/distilled water |
4g | NaOH2PO4H2O (if available) |
6g | Na2HPO4 (if available) |
Further information:
Herfort and Rawlin 1999.
Appendix 4 - Calculation of unionisedammonia
The following table (after Emerson et al. 1975) is used to calculate the amount of ammonia in freshwater that is in the toxic unionised form. When water temperature is 28°C and pH is 7.2, from the table below the percentage of total ammonia nitrogen (TAN) present in the unionised form is 1.10%. Therefore, if the concentration of TAN was 5.0 mg/L, then the amount of unionised ammonia present is 5.0 x 1.10% = 0.055 mg/L.
pH | Temperature (°C) | |||||||||
---|---|---|---|---|---|---|---|---|---|---|
12 | 14 | 16 | 18 | 20 | 22 | 24 | 26 | 28 | 30 | |
5.6 | 0.009 | 0.01 | 0.012 | 0.014 | 0.016 | 0.018 | 0.021 | 0.02 | 0.03 | 0.03 |
5.8 | 0.014 | 0.016 | 0.019 | 0.022 | 0.03 | 0.03 | 0.03 | 0.04 | 0.04 | 0.05 |
6 | 0.022 | 0.03 | 0.03 | 0.03 | 0.04 | 0.05 | 0.05 | 0.06 | 0.07 | 0.08 |
6.2 | 0.03 | 0.04 | 0.05 | 0.05 | 0.06 | 0.07 | 0.08 | 0.1 | 0.11 | 0.13 |
6.4 | 0.05 | 0.06 | 0.07 | 0.09 | 0.1 | 0.12 | 0.13 | 0.15 | 0.18 | 0.2 |
6.6 | 0.09 | 0.1 | 0.12 | 0.14 | 0.16 | 0.18 | 0.21 | 0.24 | 0.28 | 0.32 |
6.8 | 0.14 | 0.16 | 0.19 | 0.22 | 0.25 | 0.29 | 0.33 | 0.38 | 0.44 | 0.51 |
7 | 0.22 | 0.25 | 0.29 | 0.34 | 0.4 | 0.46 | 0.53 | 0.61 | 0.7 | 0.8 |
7.2 | 0.34 | 0.4 | 0.47 | 0.54 | 0.63 | 0.72 | 0.83 | 0.96 | 1.1 | 1.26 |
7.4 | 0.54 | 0.63 | 0.74 | 0.85 | 0.99 | 1.14 | 1.31 | 1.51 | 1.73 | 1.98 |
7.6 | 0.86 | 1 | 1.16 | 1.35 | 1.56 | 1.8 | 2.07 | 2.37 | 2.72 | 3.11 |
7.8 | 1.36 | 1.58 | 1.83 | 2.12 | 2.45 | 2.82 | 3.24 | 3.71 | 4.24 | 4.84 |
8 | 2.13 | 2.48 | 2.87 | 3.31 | 3.82 | 4.39 | 5.03 | 5.75 | 6.56 | 7.46 |
8.2 | 3.34 | 3.87 | 4.47 | 5.15 | 5.92 | 6.79 | 7.75 | 8.82 | 10.01 | 11.32 |
8.4 | 5.18 | 5.99 | 6.91 | 7.93 | 9.07 | 10.34 | 11.75 | 13.3 | 14.99 | 16.83 |
8.6 | 7.98 | 9.18 | 10.52 | 12.01 | 13.65 | 15.46 | 17.43 | 19.55 | 21.84 | 24.28 |
8.8 | 12.08 | 13.8 | 15.71 | 17.78 | 20.04 | 22.47 | 25.06 | 27.81 | 30.7 | 33.7 |
9 | 17.88 | 20.24 | 22.8 | 25.53 | 28.43 | 31.48 | 34.64 | 37.91 | 41.25 | 44.62 |
9.2 | 25.65 | 28.69 | 31.88 | 35.21 | 38.63 | 42.13 | 45.66 | 49.18 | 52.67 | 56.08 |
9.4 | 35.35 | 38.93 | 42.59 | 46.27 | 49.94 | 53.57 | 57.11 | 60.53 | 63.81 | 66.93 |
9.6 | 46.43 | 50.26 | 54.04 | 57.71 | 61.26 | 64.65 | 67.85 | 70.85 | 73.65 | 76.23 |
9.8 | 57.87 | 61.56 | 65.07 | 68.39 | 71.48 | 74.35 | 76.98 | 79.39 | 81.58 | 83.56 |
10 | 68.52 | 71.74 | 74.7 | 77.42 | 79.89 | 82.12 | 84.13 | 85.93 | 87.53 | 88.96 |
Appendix 5 - Water quality guidelines for the protection of cultured freshwater fish
Information presented in the table below is a summary of recommended water quality guidelines for the culture of freshwater fish, from ANZECC. (2000). Water quality guidelines for the protection of cultured fish, molluscs and crustaceans. In: Australian and New Zealand Guidelines for Fresh and Marine Water Quality. Paper No. 4 -Volume 3 (Chapter 9). (http://www.deh.gov.au/water/quality/nwqms/pubs/volume39-4.pdf). It should be noted that the values presented here do not apply equally to all situations, locations and species (ANZECC 2000).
Category | Parameter | Guideline for continuous exposure (mg/L) unless specified |
---|---|---|
Physico-chemical indicators | Alkalinity | 20-175 |
Biochemical oxygen demand (BOD) | <15 | |
Chemical oxygen demand (COD) | <40 | |
Carbon dioxide | <10 | |
Colour and appearance of water | 30-40 | |
Dissolved oxygen | >5 | |
Gas supersaturation | <100% | |
pH | 5.5-9.0 | |
Suspended solids (and turbidity) | <40 | |
Temperature | <2.0°C change over 1 hour | |
Total hardness (CaCO3) | 20-100 | |
Salinity (total dissolved solids) | <3 | |
Inorganic chemicals (heavy metals and others) | Aluminium | <0.03 (pH>6.5), <0.01 (pH <6.5) |
Ammonia (un-ionised) | <0.025 (pH>8.0) cold freshwater | |
0.0 (pH≤8.0) cold freshwater | ||
<0.3 warm freshwater | ||
Ammonia (TAN) | <1.0 | |
Arsenic | <0.05 | |
Cadmium | <0.0002-0.0018 | |
Chlorine | <0.003 | |
Chromium | <0.02 | |
Copper | <0.005 | |
Cyanide | <0.005 | |
Hydrogen sulfide | <0.001 | |
Iron | <0.01 | |
Lead | <0.001 | |
Magnesium | <15 | |
Manganese | <0.01 | |
Mercury | <0.001 | |
Nickel | <0.1 | |
Nitrate (NO3¯ ) | <50 | |
Nitrite (NO2) | <0.1 | |
Phosphates | <0.1 | |
Selenium | <0.01 | |
Silver | <0.003 | |
Inorganic chemicals(heavy metals and others) | Tributyltin | <0.000026 |
Vanadium | <0.1 | |
Zinc | <0.005 | |
Organic chemicals (nonpesticides) | Detergents and surfactants | <0.28 (LAS) |
<0.65 (AES) | ||
<0.14 (AE) | ||
Methane | <65 | |
Oils and greases (including petrochemicals) | <0.3 (petroleum) | |
Phenols | <0.0006-0.0017 | |
Polychlorinated bi phenyls (PCBs) | <0.002 | |
Pesticides | 2,4-dichlorophenol | <4.0 |
Aldrin | <0.01 | |
Azinphos-methyl | <0.01 | |
Chlordane | <0.01 | |
Chlorpyrifos | <0.001 | |
DDT | <0.0015 | |
Demton | <0.01 | |
Dieldrin | <0.005 | |
Diquat | <0.5 | |
Endosulfan | <0.003 | |
Endrin | <0.002 | |
Gunthion (see also Azinphos-methyl) | <0.01 | |
Hexachlorobenzole | <0.00001 | |
Heptachlor | <0.005 | |
Lindane | <0.01 | |
Malathion | <0.1 | |
Methoxychlor | <0.03 | |
Mirex | <0.001 | |
Parathion | <0.04 | |
Pyrethrin | <0.001 | |
Pyrethrum | <0.01 | |
Rotenone | <10 | |
Simazine | <10 | |
Toxaphene (9478) | <0.002 | |
Trichchlorphon | <0.001 |
Appendix 6 - Useful contacts
The Victorian Fisheries Authority
GPO 4440
Melbourne. VIC. 3000.
Ph: (03) 9658 4376.
Web: http://www.vfa.vic.gov.au/fishing
Aquaculture specialists
Department of Primary Industries
Primary Industries Research Victoria (PIRVic)
Private Bag 20
Alexandra. VIC. 3714.
Ph: (03) 5774 2208. Fax: (03) 5774 2659.
Department of Primary Industries
Primary Industries Research Victoria (PIRVic)
PO Box 114
Queenscliff. VIC. 3225.
Ph: (03) 5258 0111. Fax: (03) 5258 0270.
See also Victorian Aquaculture Industry: Register of Consultants (DNRE 2002) (below)
Chemicals and drugs
National Registration Authority for Agriculture and Veterinary Chemicals
PO Box E240 Kingston. ACT. 2604.
Ph: (02) 6272 5852. Fax: (02) 6272 5249.
Web: http://www.apvmqa.gov.au/
Fish health specialists
Department of Primary Industries
Primary Industries Research Victoria (PIRVic)
475 Mickleham Rd
Attwood. VIC. 3049.
Ph: (03) 9217 4354. Fax: (03) 9217 4199.
See also Victorian Aquaculture Industry: Register of Consultants (DNRE 2002) (below)
Victorian Aquaculture Industry: Register of Consultants (DNRE 2002)
http://www.vfa.vic.gov.au/fishing
The Victorian Aquaculture Industry: Register of Consultants is a reference resource that contains details of over 40 prospective aquaculture consultants Australia wide. It is intended for use by potential proponents in aquaculture and existing industry to assist in the selection of a service provider with appropriate expertise and experience. The 'Register' does not attempt to screen consultants based on their level of expertise or qualifications, rather it reproduces information gathered from a standard questionnaire in which consultants promote their own service using their own language. On this basis the ultimate judgement of worth and due diligence is left up to the purchaser / reader.
Appendix 7 - Useful Conversions
Volumes
1 m3 (cubic metre) = 1,000 L 1,000 mL (millilitres) = 1 L (litre) 1,000,000 L = 1 ML (megalitre)
Weight
1,000 ug (micrograms) = 1 mg (milligram) 1,000 mg = 1 g (gram) 1,000 g = 1 kg (kilogram) 1,000 kg = 1 tonne
Conversions
1 ppm (parts per million) = 1 mg/L (milligram per litre)
1 ppm = 1 L in 1,000,000 L
1 ppm = 1 mL in 1,000 L
1ppt (parts per thousand) = 1 g/L (gram per litre)
1 ppt = 0.1%
1 ppt = 1,000 ppm
1% = 10ppt
1% = 10 g/L
1 mg/L= 1 g in 1,000 L
Appendix 8 - Useful internet sites
Federal Government
Aquaplan. Australia's National Strategic Plan for Aquatic Animal Health 1998-2003. National Office of Animal and Plant Health, Agriculture, Fisheries and Forestry - Australia, Canberra. 33 pp. www.affa.gov.au.
Australian Pesticides and Veterinary Medicines Authority (APVMA). Lists information on drugs and chemicals registered for use in aquaculture in Australia.
http://www.apvmqa.gov.au
Australian and New Zealand Guidelines for Fresh and Marine Water Quality. Water quality guidelines for the protection of cultured fish, molluscs and crustaceans. http://www.deh.gov.au/water/quality/nwqms/pu bs/volume3-9-4.pdf
Disease Watch. latest information on training and awareness resources for aquatic animal disease emergencies. http://www.diseasewatch.com
Victorian State Government
The Victorian Fisheries Authority, Department of Primary Industries.
http://www.vfa.vic.gov.au/fishing
Other
Aqualink Aquarium Service. Common Freshwater Diseases. A glossary of common diseases which infect freshwater fish. Although not every disease is treatable--or easily diagnosed, this list should serve as a basic aid in diagnosing ailments.
http://www.aqualink.com/disease/sdisease.html
Aquaculture Health Page. Fish disease information.
http://www.geocities.com/CapeCanaveral/Lab/74 90/#fishdis
FDA Drugs Approved for Use in Aquaculture(Poikilothermic Food Species) in the USA.
http://www.fda.gov/cvm/index/aquaculture/app endixa6.htm
FINS: The Fish Information Service. Fish Disease Diagnosis.
http://fins.actwin.com/disease/chart1.php
Fish-Disease.com. Information on nearly 70 different diseases and over 200 different Medications, their active ingredients, their purpose, and how to administer them. Interactive Disease Diagnostic Center designed to help determine the illness affecting fish.
http://www.fish-disease.com/fish-disease.htm
Microscope Diagnosis of common parasite infections of fish.http://www.ntlabs.co.uk/microscope_diagnosis.htm
Aquatic Disease. A Veterinary Medical Primer.http://www.athiel.com/Lib10/drj4.htm
A discussion board for fish health issues.http://www.oceanproaquatics.com/cgibin/board/index.cgi
A broad range of fish health topics.http://www.koivet.com/
A site dedicated to the aquarium hobbyist and fish enthusiast, with a small section on fish health
http://www.algone.com/fish_health.htm
Aquatic Diagnostic Services International, Phone: 073855 2333, Mobile: 0414 478 630, Fax 07 3354 2149, Email: fishdoc@adsi.com.au Web: www.adsi.com.au
Appendix 9 - Glossary
Abiotic | Non-living. |
Anaerobic | requiring the absence of oxygen (eg. anaerobic bacteria) |
Bacterivore | Bacteria-eating. |
Biocides | Substances that are capable of killing living organisms. |
Biopsy | Excision and diagnostic study of tissue from a living body. |
Biotic | Pertaining to life or living organisms. |
Cilia | Minute, fine, hair-like outgrowths |
Commensal | An organism living with another and sharing food, both species as a rule benefiting by the association, or one benefiting and the other not being harmed. |
Contractile | Capable of contracting. |
Cosmopolitan | World-wide in distribution |
Cyst | Protective, spore-like cell; an enclosed membrane |
surrounding an organism or group of organisms. | |
Denticles | Small tooth-like processes. |
Dyspnoea | difficult or laboured breathing. |
Facultative | Ability to live either a parasitic or non-parasitic life. |
Filter-feeding | Feeding on small organisms by straining them out of the surrounding medium. |
Flagella | Long, whip-like projections. |
Haemorrhagic | Characterised by discharge of blood. |
Hyphae | Thread-like filaments of a fungus. |
Hypoxia | State where insufficient oxygen is available for utilisation by tissues. |
Lesion | An alteration to the normal structure of cells, tissues or organs. |
Metazoan | Multicellular organisms. |
Necrosis | Death of a particular part of a tissue or organ. |
Obligate | With no choice. An obligate parasite depends on its host to live. |
Opercula | External covering of gills. |
Osmoregulation | Regulation of the osmotic pressure in the body by controlling the amount of water and/or salts in the body. |
Posterior | Pertaining to, or situated toward, the rear end. |
Protozoan | Microscopic organism; usually single celled but may consist of a group of similar cells. |
Septicaemia | Invasion and persistence of pathogenic bacteria in the bloodstream. |
Ubiquitous | Being everywhere. |
Ulcer | Break in the skin or surface membrane, with disintegration and necrosis of tissue. |
μm | micrometres, 0.001 mm. |
Visceral organs | Organs within the body cavity. |
Brett A. Ingram, Fiona Gavine and Peter Lawson (2005)
Preferred way to cite this publication:
Fish Health Management Guidelines for Farmed Murray Cod. Fisheries Victoria Research Report Series No. 32.
ISSN 1448-7373
ISBN 1 74146 678 4
Author Contact Details:
Brett Ingram
Primary Industries Research Victoria
Private Bag 20,
Alexandra
Victoria 3714.